DOI:
10.1039/D4RA06626J
(Review Article)
RSC Adv., 2024,
14, 31777-31796
Bimetallic metal–organic frameworks (BMOFs) for dye removal: a review
Received
13th September 2024
, Accepted 3rd October 2024
First published on 8th October 2024
Abstract
Safe drinking water and a clean living environment are essential for good health. However, the extensive and growing use of hazardous chemicals, particularly carcinogenic dyes like methylene blue, methyl orange, rhodamine B, and malachite green, in both domestic and industrial settings, has led to a scarcity of potable water and environmental challenges. This trend poses a serious threat to human society, sustainable global development, and marine ecosystems. Consequently, researchers are exploring more advanced methods beyond traditional wastewater treatment to address the removal or degradation of these toxic dyes. Conventional approaches are often inadequate for effectively removing dyes from industrial wastewater. In this study, we investigated bimetallic metal–organic frameworks (BMOFs) as a solution to these limitations. BMOFs demonstrated outstanding dye removal and degradation capabilities due to their multifunctionality, water stability, large surface area, adjustable pore size, and recyclability. This review provides a comprehensive overview of research on dye removal from wastewater using BMOFs, including their synthesis methods, types of dyes, and processes involved in dye removal, such as degradation and adsorption. Finally, the review discusses the future potential and emerging opportunities for BMOFs in sustainable water treatment.
1. Introduction
Persistent organic pollutants are commonly found in the waste products of the chemical, dyeing, pharmaceutical, and paper industries, posing a significant environmental pollution challenge. As a result, there is an urgent need for cost-effective and efficient methods to manage and reduce these pollutants.1,2 The rapid expansion of the global population, climate change, and industrial progress have significantly impacted water quality, contributing to a growing global freshwater crisis. In this context, various users and polluters of freshwater play a major role in depleting this essential resource.3–5 Notably, fabric dyeing is one of the primary contributors to water pollution, with textile dyeing ranking as the second-largest source of water contamination worldwide.6 Among the most frequently used dyes, substances like MB,7 RhB,8 MO,9 CR,10 MR,11 and CV are prominent industrial pollutants originating from diverse sectors such as textiles,12,13 cosmetic,14 leather,15 food,16 pharmaceutical,17 paint and varnish,18,19 and pulp20 and paper industries.21 As shown in Fig. 1, a recent estimate indicates that approximately 7 × 108 kg of dyes are produced each year. However, due to inefficiencies in the dyeing processes, the textile industry contributes up to 7 × 108 kg of these dyes to wastewater annually during dyeing and finishing operations.22 Therefore, it is crucial to remove dyes from wastewater.
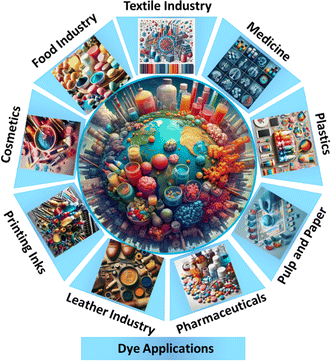 |
| Fig. 1 Different types of dyes and their potential industrial applications. | |
Several methods have been employed to remove dye pollutants to date, including ozonation,23 filtration,24 bioadsorption,25,26 biofilm reactors,27,28 electrocoagulation,29 ion exchange removal,30,31 adsorption,32,33 catalytic reduction,34,35 photocatalytic degradation,36,37 and biological/aerobic.38,39 The use of various advanced material applications is among the most effective approaches for dye removal. Significant progress in nanomaterials, such as metal oxides,40–43 carbon dots,44–51 sulfur dots,52 and metal–organic framework (MOF)-based nanoparticles,53–55 has been notable in the early 21st century. These synthesized materials have proven successful in environmental treatment and protection. Notably, MOF-based materials are the most widely used in this domain due to their cost-effectiveness, diverse configurations and structures, high thermal and mechanical stability, adjustable pore properties, extensive surface area, and reusable metal sites.56–61
MOFs have recently attracted considerable attention for their photocatalytic properties. These functional hybrid materials are formed by connecting organic ligands with metal ions, and they offer a variety of advantageous features, including high porosity, large surface area, exposed metal sites, and the flexibility to be customized through various material combinations and synthesis techniques.62–64 These qualities make MOFs highly useful in a wide range of applications, including sensing,65,66 catalysis,67–70 drug delivery,71–73 pollutant removal from water,74,75 and energy storage or conversion.76
BMOFs are created by linking two distinct metal ions with an organic ligand.77 Although metal substitutions are commonly used in the chemistry of oxides and intermetallic compounds, this approach has been less frequently applied to MOF production, likely because metallic centers are generally more associated with purely inorganic materials than with hybrid ones.78 Nonetheless, bimetallic MOFs have recently gained widespread interest due to their outstanding structural and chemical stability, significant porosity, and potential applications in gas adsorption,79 separation,80 catalysis,81 sensing,82,83 and biochemistry.84 Thanks to their high porosity and numerous adsorption sites, BMOFs have demonstrated advantages over monometallic MOFs in various applications. They also show promise as precursors or templates for the development of BMOF-derived photocatalysts, which possess more active sites and enhanced conductivity compared to their monometallic counterparts.85 As a result, the use of BMOFs for dye removal holds great promise and is highly significant for environmental applications.
Several research groups have investigated various methods for addressing dye-contaminated wastewater using different materials.86–91 This literature review provides an overview of the use of BMOFs for removing dye pigments from wastewater, emphasizing the effectiveness of BMOFs as adsorbents compared to other materials in dye removal. Additionally, the review explores the mechanisms of BMOF-dye adsorption and the future prospects for using BMOFs in this application. To our knowledge, no existing review paper offers a comprehensive discussion on dye removal specifically with BMOFs. Thus, this review aims to present the latest information on the application of BMOFs for dye removal from aqueous solutions, along with future perspectives, as illustrated in Fig. 2.
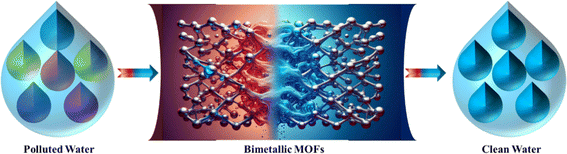 |
| Fig. 2 Schematic diagram depicting BMOFs for dye removal. | |
1.1 Categorization of dyes
Dyes are commonly classified based on their structural or functional groups, color, and ionic charge when dissolved in water.92 Since the ionic classification of dyes significantly impacts adsorption efficiency, this review adopts that classification. As illustrated in Fig. 3, dyes are divided into ionic and non-ionic categories. Non-ionic dyes include vat and disperse dyes, while ionic dyes are further categorized as cationic (basic) and anionic (direct, acidic, and reactive).93
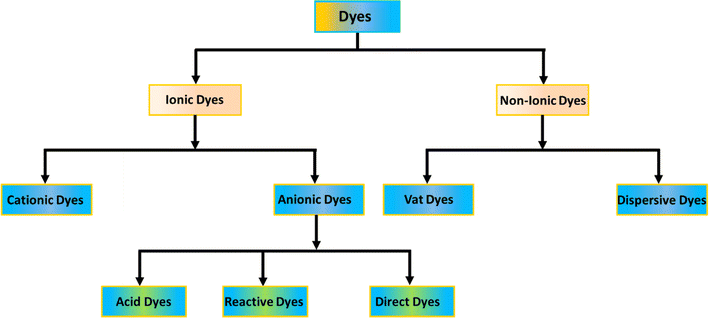 |
| Fig. 3 Categorization of dyes. | |
The components of dyes make them toxic. Typically, the presence of dyes in water bodies can affect the photosynthesis of aquatic life by blocking sunlight transmission. More concerning is that many dyes are mutagenic, carcinogenic, or teratogenic to both animals and humans. Dye molecules in wastewater are known to cause dysfunction in multiple human organs. Direct, cationic, acidic, and disperse dyes can all contribute to the development of benign and malignant tumors, with direct dyes being linked to bladder cancer. Reactive dyes can cause dermatitis, rhinitis, allergic conjunctivitis, and occupational asthma. Additionally, many of the dyes discussed are carcinogenic, highlighting the importance of effectively treating dye-contaminated wastewater from dye manufacturing plants.94 As shown in Fig. 4.
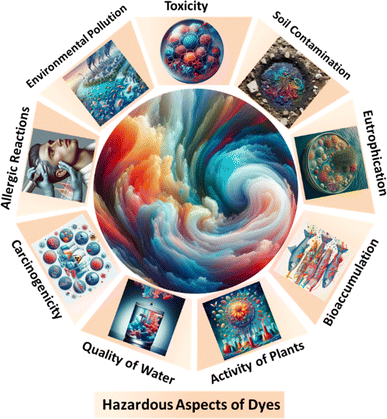 |
| Fig. 4 Hazardous aspects of dyes. | |
2. Mechanism for dye removal
The adsorption of molecules on the surface of an adsorbent can occur in two distinct ways, depending on the interaction between the solid surface (BMOFs) and the adsorbed molecules: physical and chemical sorption. In physisorption, electrostatic interactions and van der Waals forces are involved, making the process reversible. A possible mechanism is illustrated in Fig. 5. The adsorption mechanism on the adsorbent surface involves three types of interactions: (1) electrostatic interactions, (2) hydrogen bonding, and (3) π–π stacking interactions, all contributing to the enhanced adsorption of dye molecules. In contrast, chemisorption involves strong covalent bonds as the primary interaction between the adsorbent and adsorbate, leading to diffusion from the surface into the material's interior, typically forming a monolayer.
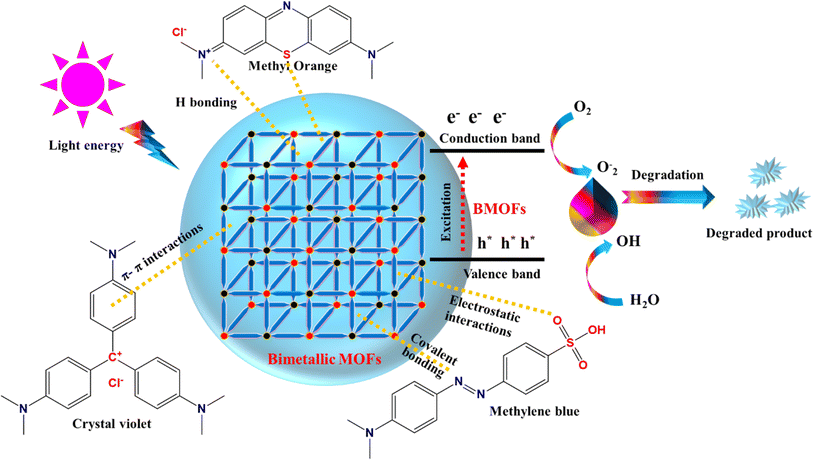 |
| Fig. 5 Schematic diagram of dye adsorption and degradation. | |
In recent years, the use of biological sources for dye degradation has emerged as a promising and eco-friendly alternative to traditional chemical methods for removing dyes from polluted water and soil. This approach harnesses natural biological processes, such as microbial activity and enzymatic reactions, which can break down harmful dye compounds without generating toxic byproducts. Alongside biological methods, researchers have focused on developing engineered BMOFs for enhanced dye degradation. These BMOFs are synthesized from various metal combinations and organic linkers, offering a unique structure with high porosity and active sites that facilitate the breakdown of complex dye molecules.
One particularly promising method is the photocatalytic degradation of dyes using BMOFs. Under light irradiation, BMOFs can generate reactive species, such as hydroxyl radicals and superoxide anions, which play a crucial role in oxidizing and decomposing dye molecules. The presence of two different metals in the BMOF structure enhances light absorption and charge separation, making the photocatalytic process more efficient. This process, referred to as dye degradation by BMOFs in the presence of light, is illustrated in Fig. 5, showing how these materials can harness light energy to degrade dyes into less harmful compounds.
3. BMOF properties, and synthesis
Although single-metal MOFs offer a broad range of structural compatibilities, using transition metals (Fe, Zn, Co, Ni) in BMOFs can lower costs and improve catalytic performance.95,96 BMOFs can be categorized into two types based on their spatial arrangement: different metals in separate secondary building units (SBUs) or different metals within the same SBUs. The latter typically exhibits greater catalytic activity due to its denser structure, improved stability, and enhanced electron transfer.97 Combining two metal cations can boost conductivity and promote oxidation reactions between different metal sites within the MOF, leading to higher catalytic efficiency. This method of integrating functional components can create multifunctional complexes with superior properties, enhancing activity for redox reactions, supercapacitors, and other processes.98 Although BMOFs are still under development, increasing research highlights their promising potential for various practical applications.
BMOF-based composites offer several benefits compared to monometallic MOFs: (i) they combine different metallic elements, organic ligands, and structures from monometallic MOFs, leading to a wide range of compositions and functions; (ii) they enhance pore development, with their synthesis being relatively simple and gentle; (iii) their structured arrangement of metal ions and ligands improves the fixation, dispersion, stability, and catalytic activity of the materials.99,100 Considering these benefits, numerous studies have documented nanomaterials based on BMOFs with diverse compositions and structural properties that are extensively employed in environmental pollution management.101,102
3.1 Advantages of bimetallic MOFs over monometallic MOFs
Bimetallic MOFs present numerous advantages over their monometallic counterparts, particularly in terms of enhanced stability. The interaction between two distinct metal ions in BMOFs leads to improved structural integrity. As a result, they typically exhibit superior thermal, chemical, and mechanical stability compared to monometallic MOFs, which is crucial for withstanding extreme conditions such as high temperatures, acidic or basic environments, and varying pressures.99,103,104 Characterized by their high porosity and abundance of adsorptive sites, BMOFs outperform monometallic MOFs in several applications.105 They also hold promise as precursors or templates for developing BMOF-derived photocatalysts, which offer a larger number of active sites and greater conductivity than their monometallic-derived counterparts.106
Moreover, the inclusion of two metals allows for more precise tuning of a MOF's properties, such as pore size, electronic structure, and adsorption capabilities. This ability to customize these properties enhances performance.107 The synergistic effects between the metals can result in improved redox behavior, better conductivity, and increased adsorption capacity. The dual-metal system also facilitates the adjustment of electronic, magnetic, and optical properties, making BMOFs highly versatile and suitable for a wider range of applications.108–110
3.2 Synthesis of BMOFs
Various methods have been developed for the synthesis of MOFs, including hydrothermal,111 solvothermal,112 microwave,113 electrochemical,114 sonochemical,115 and reflux techniques.116 Several of these techniques can also be used to synthesize bimetallic MOFs. Typically, synthesis methods fall into two main categories: one-pot synthesis and post-synthetic modification117 (Fig. 6). Unlike the synthesis of monometallic MOFs, the synthesis of BMOFs requires strict control of nucleation rates and growth kinetics due to the differing reaction kinetics of the two types of metal ions or clusters.
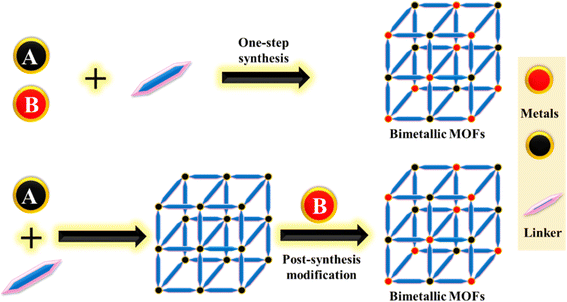 |
| Fig. 6 Illustration of synthesis strategies for BMOFs using one-step and post-synthetic approaches. | |
3.2.1 Direct, and solution based synthesis. BMOFs can be created by combining metal salts using a solvothermal process. However, simply mixing different metal salts does not guarantee the formation of BMOFs.118 To avoid the formation of mixed phases and achieve a specific structure, it is important to control factors such as solubility, molar ratios of metal ions, and pH. One promising approach for creating BMOFs with a defined composition involves synthesizing predefined secondary building units (SBUs) as precursors for the metal nodes.119Several bimetallic or bi-ligand MOFs are solid solutions with adjustable ratios of ligands or metals. These BMOFs can be created directly by employing multiple ligands or metals, or through post-synthetic modification. The term “solid solution” is used because these MOFs do not have a completely random arrangement of ligands or metals.120,121
3.2.2 One pot synthesis (OPS). The OPS method, also known as ‘one-pot’ synthesis, has significantly improved the process of integrating secondary metal centers into MOFs for various applications.122 BMOFs can be created by mixing both metal salts in the same reaction mixture, leading to structures that contain two metal species. This method simplifies the synthesis process and reduces the need for intermediate steps. MOFs with these additional metal nodes often exhibit complex and delicate networks, enhancing their potential applications due to unique synergistic effects. The success of this synthesis relies on selecting the right precursors, optimizing reaction conditions, and carefully adjusting parameters to produce high-quality BMOFs.103,123
3.2.3 Post synthetic modification method (PSM). Another effective method for fabricating BMOFs is post-synthetic modification (PSM). This approach allows for either the exchange of pre-synthesized metal clusters or organic ligands in a MOF or for altering the MOF structure to introduce secondary metal nodes. The success of PSM depends on the stability, porosity, and crystallinity of the initial structure. PSM mainly involves the exchange of metal ions and the elimination–addition of metals.103 One of the main challenges is to precisely control the ratio of the two types of metal sites in BMOFs across a broad range.The first approach, metal ion exchange, is a highly effective method for synthesizing BMOFs. In this method, monometallic (M) MOFs are typically placed into a solution containing secondary metal ions with similar properties, facilitating ion exchange with the metal nodes in the framework. The degree of exchange can be controlled by adjusting experimental parameters.124 In summary, BMOFs can be synthesized via ion exchange using two types of metal ions with similar properties.
The second strategy, metal elimination–addition, involves the sequential removal of some metal sites in monometallic MOFs to create vacancies, which are then filled with different metal ions. The new metal ions should have a similar charge and coordination mode to those of the ions being replaced.125,126
3.2.4 Template method. A method involving templates can effectively regulate the composition of metal ions in BMOFs. Furthermore, this synthesis approach can be used to create hollow BMOFs, offering benefits such as increased active sites and improved mass transport. There are two main approaches to this synthesis method: the self-template method and the exterior-template method. The self-template method is a straightforward way to produce hollow BMOFs, as there is no need to remove the template; the process involves dissolution-regrowth. In contrast, the exterior-template method utilizes a sacrificial template that must be removed after synthesis.119
3.2.5 Core–shell (CS) BMOFs. In core–shell (CS) BMOFs, the outer shell and inner core are constructed using different metal centers. Seed-induced growth has been proven to be an effective method for producing core–shell nanomaterials. Through epitaxial growth, two MOFs with similar lattice parameters can be combined to form CS MOFs.127 Another method, post-synthetic selective exchange of metal ions within the framework, can also be utilized to create CS BMOFs. This is possible because the metal sites in the core and near the surface of the MOF exhibit different flexibilities and, consequently, distinct reactivities. Therefore, by carefully controlling the post-synthetic metal exchange process, CS BMOFs can be produced through selective transmetalation.108
4. Dye removal by BMOFs
Rapid industrial expansion is a significant contributor to water pollution.128 Among the various pollutants present in water bodies, organic dyes are particularly problematic, as they pose serious risks to both humans and animals. A substantial portion of industrial wastewater consists of dye-contaminated effluents.129 Globally, approximately 800
000 tons of dye are produced each year, with nearly 20% of these effluents being released into the environment regularly without adequate public or environmental awareness.130 Therefore, it is crucial to handle these corrosive dyes with care. Numerous porous adsorbents have been investigated for their effectiveness in removing dyes from water.131–135 Among these, BMOF-based porous materials exhibit remarkable capabilities for dye removal in aqueous environments.
The following sections will explore recent advancements in BMOFs for the removal of both cationic (C) and anionic (A) dyes from water samples. Dyes can be categorized into three groups: cationic (C), anionic (A), and nonionic, each encompassing numerous types of dyes. Anionic dyes include direct, reactive, and acid dyes, while basic dyes represent cationic dyes, and dispersed dyes represent nonionic dyes.136 For greater efficiency, the effect of pH must be considered. The initial pH of the dye solution significantly influences factors such as ionization levels, surface charge density, and the adsorption capacity of the adsorbent, playing a crucial role in the overall adsorption process. The adsorption rate fluctuates with changes in the pH of the medium. At low pH levels, the removal efficiency of cationic dyes decreases, while for anionic dyes, it increases due to the highly protonated surface of the adsorbent, which favors the adsorption of anionic groups and enhances the overall process. However, at high pH levels, the opposite effect is observed.137
4.1 Removal of cationic (C) dyes
Cationic (C) dyes in water carry a positive charge, are highly soluble, and exhibit strong coloration even at low concentrations. These dyes can be readily and effectively adsorbed onto the negatively charged surfaces of adsorbents through electrostatic attraction. However, the efficiency of removal can vary based on the surface area and the functional groups available on the adsorbent's surface.
4.1.1 Methylene blue removal. Methylene blue (MB) is frequently utilized as a benchmark dye in microfiltration and adsorption research due to its molecular characteristics, which make it suitable for various applications, particularly in the medical field. Nevertheless, if not properly treated before disposal, it can pose environmental risks. Studies have shown that overexposure to MB can lead to methemoglobinemia by directly oxidizing hemoglobin. Additionally, it has the potential to induce issues related to hemolysis, particularly in newborns. Prolonged exposure to methylene blue may ultimately result in significant anemia.138 Hence, the hazardous and highly pigmented MB dye needs to be eliminated from wastewater prior to its release into the environment.This study by Eltaweil et al.139 introduces a new composite as an effective adsorbent for cationic MB dye. The UiO-66/MIL-101(Fe) BMOF was created using a solvothermal method. The adsorption capability of the UiO-66/MIL-101(Fe)–GOCOOH composite was evaluated using a batch technique, showing that it had a higher adsorption capacity compared to the BMOF alone. Adsorption isotherms and kinetic studies indicated that MB dye adsorption on the composite follows the Langmuir isotherm model and both pseudo-first-order and pseudo-second-order kinetic models. Additionally, thermodynamic data suggested that the adsorption process is endothermic, spontaneous, and involves both physisorption and chemisorption. The newly developed composite also demonstrated good reusability, making it a highly promising adsorbent for efficiently treating dye-containing industrial effluents. As shown in Fig. 7.
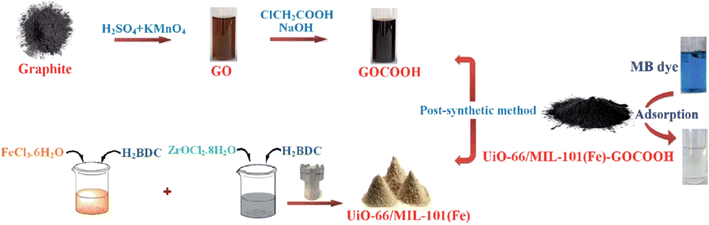 |
| Fig. 7 Diagram showing the synthesis of the composite and images illustrating the adsorption process. From ref. 139 with permission. Copyright 2020, Royal Society of Chemistry. | |
Shan et al.140 synthesized a series of MOF materials using a solvothermal method, with trimellitic acid and terephthalic acid reacting with nickel and cobalt metal salts for the photodegradation of MB under xenon light irradiation. Among these, ML-3, a bimetallic mixed-ligand MOF material with a specific ratio and a tight-flower structure based on the solid block structure NC-3, exhibited outstanding degradation performance against MB. While NC-3 achieved a degradation efficiency of 80.6% in 120 minutes, ML-3 reached 97.8% in the same timeframe. Radical trapping experiments and Mott–Schottky analysis revealed that h+ and ˙O2− were the primary active substances in the photocatalytic degradation mechanism. The study found that the redox potential of MB (1.77 eV) was lower than the valence band (VB) potential of ML-3 (2.01 eV), allowing h+ to directly oxidize MB. Consequently, the materials synthesized in this study are effective for wastewater treatment and show significant potential for environmental applications, as shown in Fig. 8.
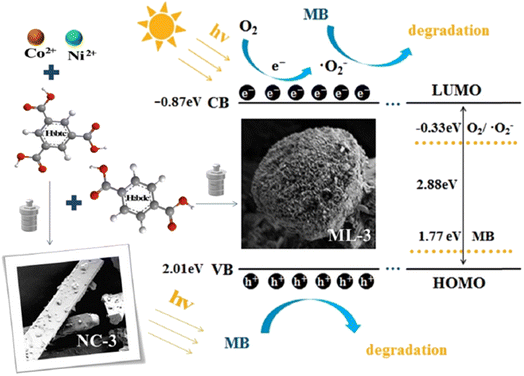 |
| Fig. 8 Diagram of sample synthesis and photocatalytic mechanism. Form ref. 140 with permission. Copyright 2022, Elsevier. | |
Ma et al.141 synthesized Ce/Co BMOFs. The photocatalytic activity was tested under Xe lamp irradiation with MB as a model pollutant. Among the samples, DT-3, with a Ce/Co ratio of 4
:
1 and an H2bdc/H3btc ratio of 1
:
2, demonstrated the highest photocatalytic performance, achieving a 97.6% degradation efficiency of MB within 120 minutes. Analysis revealed that DT-3 had a bandgap energy of 3.20 eV, with a conduction band potential of −0.75 eV, which is more negative than the standard redox potential of O2/˙O2−, and a VB potential of 2.45 eV, exceeding the redox potential of MB. Overall, the results indicate that the synthesized bimetallic photocatalyst with mixed ligands is effective in degrading the MB dye in wastewater. Table 1 summarizes studies that utilized BMOFs for the removal of MB.
Table 1 Degradation of MB by BMOFs
Catalysts |
Method |
Catalyst dosage |
Initial concentration |
Temperature °C |
pH |
Performance% |
Ref. |
Zn/Co MOFs |
Electrosorption |
— |
2847 mg g−1 |
— |
— |
90 |
142 |
Zn/Co MOFs |
Degradation |
0.01 g L−1 |
128 mg L−1 |
— |
— |
40 |
143 |
Fe/CoMIL-88B |
Degradation |
0.8 g L−1 |
0.1 mM |
70 |
10 |
100 |
144 |
Al/Cu MOFs |
Adsorption |
0.1 g L−1 |
381.68 mg g−1 |
25 |
4–6 |
84 |
145 |
Zn/Mn MOFs |
Degradation |
1 g L−1 |
10 mg L−1 |
90 |
— |
91.23 |
146 |
Ni/Co MOF@MAC |
Adsorption |
0.2 g |
40 mg L−1 |
25 |
6 |
— |
147 |
Co/Fe MOFs |
Adsorption |
1 g L−1 |
1 mg L−1 |
90 |
4 |
90 |
53 |
Ni/Zn MOFs |
Degradation |
40 mg |
10 mg L−1 |
— |
— |
97.4 |
148 |
Cu/Co MOFs |
Degradation |
0.6 g L−1 |
— |
45 |
6.2 |
93.29 |
149 |
Cu/Co MOFs |
Degradation |
50 mg L−1 |
0.2 mM |
150 |
7.15 |
100 |
150 |
Fe/Cu MOFs |
Degradation |
0.6 g L−1 |
0.2 mM |
25 |
9.05 |
100 |
151 |
Co/Ni MOFs |
Degradation |
0.16 g |
10 mg L−1 |
— |
5 |
99 |
152 |
Cu/Zn MOFs |
Adsorption |
5 g L−1 |
200 mg L−1 |
25 |
7 |
98 |
153 |
Ti/Zr MOFs |
Degradation |
10 mg |
20 mg L−1 |
— |
— |
93.2 |
154 |
Co/Ni MOFs |
Degradation |
150 mg L−1 |
20 mg L−1 |
20 |
6.23 |
99.4 |
155 |
Co/Ni-MOFs@BiOI |
Degradation |
0.3 g L−1 |
20 mg L−1 |
— |
5 |
81.3 |
156 |
Ni/Co MOFs |
Degradation |
8 mg |
10 mg L−1 |
— |
— |
100 |
58 |
Zn/Co ZIFs |
Degradation |
1 mg |
10 mg L−1 |
— |
Neutral |
45 |
157 |
Fe/Co MOFs |
Degradation |
20 mg |
20 mg L−1 |
— |
— |
— |
158 |
Tb/Eu MOFs |
Adsorption |
20 mg |
5 × 10−5 M |
— |
— |
99 |
159 |
4.1.2 Rhodamine B (RhB) removal. RhB is a water-soluble organic dye commonly used to color wool, cotton, silk, paper, and fabrics.160 However, RhB contamination in water can be toxic to plants and carcinogenic to living organisms due to its aromatic structures.161 Therefore, removing RhB from industrial wastewater before it is released into the environment is essential.RhB dyes were effectively removed using a new bimetallic Co/Fe-MOF developed by Hu et al.162 This innovative MOF exhibits photocatalytic activity for degrading RhB. With a catalyst dosage of 200 mg L−1, a degradation efficiency of 99.7% was achieved within 30 minutes at a temperature of 25 °C under visible light irradiation.
Xiao et al.163 synthesized cobalt-doped MIL-101(Fe) to enhance the catalytic performance of MIL-101. By optimizing the cobalt doping ratio, MIL-101(Fe,Co) was identified as having the highest catalytic activity. The activation performance of BMOFs for peroxymonosulfate (PMS) was tested using RhB as a model pollutant under the following conditions: [RhB] = 10 mg L−1, [catalyst] = 0.2 g L−1, and [PMS] = 0.4 g L−1, with a reaction time of 15 minutes. The results demonstrated that more than 99% of RhB was degraded within 15 minutes, and the catalyst maintained effective degradation across a broad pH range of 3–11. Additionally, MIL-101(Fe,Co) exhibited excellent stability, retaining over 90% degradation efficiency even after five cycles. As shown in Fig. 9.
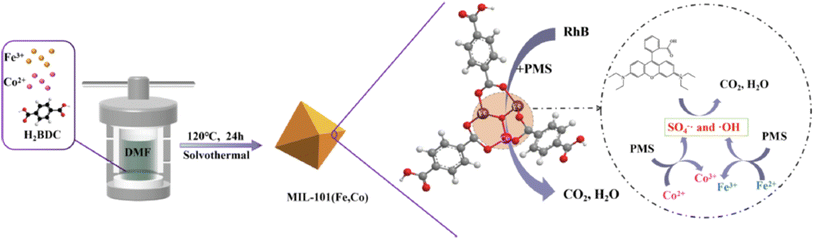 |
| Fig. 9 The schematic shows the synthesis of MIL-101(Fe,Co) and its dye degradation mechanism. From ref. 163 with permission. Copyright 2023, Elsevier. | |
A new Cd/Zr-MOF was successfully synthesized. The study examined how the Cd/Zr molar ratio and temperature influence the structure of the Cd/Zr-MOF and its photocatalytic efficiency in degrading RhB under simulated sunlight. The findings revealed that the Cd/Zr-MOF with a 5
:
1 Cd/Zr molar ratio, synthesized at 160 °C, demonstrated the highest photocatalytic performance, achieving a 95.82% degradation efficiency after 105 minutes of irradiation, significantly surpassing the efficiency of pure Cd-MOF.164
Table 2 summarizes studies that utilized BMOFs for the removal of RhB.
Table 2 Degradation of RhB by BMOFs
Catalysts |
Method |
Catalyst dosage |
Initial concentration |
Temperature °C |
pH |
Performance% |
Ref. |
Fe/Al MOFs |
Degradation |
0.10 g/100 mL |
10−4 M |
— |
— |
99.61 |
165 |
Zn/Ru MOFs |
Reduction |
2 mg mL−1 |
5.0 mM |
— |
— |
99 |
166 |
La/Fe MOFs |
Degradation |
1 g L−1 |
10 mg L−1 |
— |
— |
96 |
167 |
Fe/Co MOFs |
Degradation |
0.3 g L−1 |
20 mg L−1 |
— |
4.7 |
98.7 |
168 |
Co/Fe MIL88/MCC |
Degradation |
60 mg L−1 |
5 mg L−1 |
— |
5.5 |
87 |
169 |
Fe/Ni MOFs |
Degradation |
5 mg |
20 mg L−1 |
— |
7 |
100 |
170 |
Fe/Cu MOFs |
Degradation |
1.19 g L−1 |
10 mg L−1 |
— |
Neutral |
80.92 |
171 |
Bi/Zn MOFs |
Degradation |
0.5 g L−1 |
10 mg L−1 |
— |
— |
99 |
172 |
Ni/Co-MOF@GNS |
Degradation |
0.05 mg mL−1 |
25 mg L−1 |
— |
11 |
94 |
173 |
Cu/Fe MOFs |
Degradation |
0.25 g L−1 |
10 mg L−1 |
|
Neutral |
92 |
174 |
M/Fe MOFs |
Degradation |
5 mg |
3 10−5 M |
— |
— |
85–92 |
175 |
M/Fe MOFs |
Degradation |
9 mg |
3 10−5 mol L−1 |
— |
5 |
96 |
176 |
4.1.3 Other cationic dye.
Crystal violet (CV). The CV dye is a vibrant triphenylmethane dye commonly utilized in the textile, dyeing, leather production, printing, and food sectors. It poses health risks, as it is carcinogenic, teratogenic, and mutagenic, even in minimal concentrations.177 Jais et al. introduced a new material, NiFe-MOF@AHC, designed to effectively remove large-sized pollutants, such as CV dye. The composite showed rapid removal of CV, primarily through chemisorption. The maximum adsorption removal (Qmax) of the composite for CV dye (395.9 mg g−1) was 1.5 times lower than that of AHC. Regeneration studies indicated that the removal efficiency decreased after the first cycle but remained consistent until the fourth cycle, suggesting that the solvothermal growth of NiFe-MOF on AHC successfully produced a stable and reusable adsorbent.178
Methyl violet (MV). Methyl violet (MV) holds significant importance due to its wide-ranging applications in textiles, paints, and printing inks. It is commonly used for dyeing materials.179 In the biomedical field, MV serves as the active ingredient in Gram's stain, which is used for bacterial classification. Additionally, it can be employed as a moderate-level disinfectant, although it has been found to be toxic to most animals. Inhalation of MV may irritate the respiratory tract, while ingestion typically leads to gastrointestinal irritation.180 Prolonged or repeated exposure to methyl violet (2B) may cause damage to specific organs.181,182 Hence, it is crucial to eliminate this dye from wastewater before releasing it into water bodies.Thu and colleagues developed BMOFs (FeZn-ZIFs) that were utilized as a heterogeneous catalyst to remove methyl violet 2B dye from an aqueous solution. Under the catalytic conditions of a catalyst dosage of 0.3 g L−1, an initial dye concentration of 20 mg L−1, and at room temperature, the FeZn-ZIFs demonstrated a 95% removal efficiency of MV.183
4.2 Anionic (A) dyes
A dyes rely on a negative ion. This category encompasses various dye compounds from different classes, including azoic, anthraquinone, triphenylmethane, and nitro dyes. Despite their structural differences, these dyes share water-solubilizing ionic substituents. Direct dyes are also part of the A dye category, with a significant portion of reactive dyes falling under the group of A azo dyes from a chemical perspective.137
4.2.1 Methyl orange (MO). MO is classified as an A dye that finds widespread application as a pH indicator and is also utilized in industries related to paper and dyeing.184 Like other dyes, methyl orange is considered toxic and potentially carcinogenic.185 Contact with this dye can lead to symptoms such as diarrhea and vomiting.186 Researchers have explored the use of various agricultural waste materials for the removal of methyl orange dye.Tang et al. introduced a series of low-crystalline Fe/Ce-MOFs synthesized using DBD plasma technology as promising photocatalysts. These materials demonstrated outstanding photocatalytic performance, achieving a 93% degradation rate of MO (20 mg L−1) within 30 minutes under visible light. This cost-effective and straightforward approach offers potential advantages for the development of other low-crystalline BMOFs with superior performance.187
A different study assessed the effectiveness of the Mn/Zn@ZIF-8 nanocomposite in adsorbing MO dye from water. The adsorption isotherm analysis indicated that MO adsorption on Mn@ZIF-8 follows a monolayer pattern, aligning with the Langmuir isotherm. The Mn@ZIF-8 nanocomposite achieved a remarkable qmax of 406 mg g−1, which is notably higher than that of pure ZIF-8. Furthermore, the synthesized Mn@ZIF-8 material demonstrated strong reusability, maintaining up to 92% of its adsorption efficiency after four cycles compared to the initial cycle. Overall, the Mn/Zn@ZIF-8 nanocomposite is a promising candidate for treating industrial wastewater contaminated with MO.188
Finally, ZIF-67 and Ni-doped ZIF-67 were selected for their high stability in aqueous environments, porosity, and ease of synthesis. A porous Ni-doped ZIF-67 nanocomposite was created by incorporating nickel (Ni) into the ZIF-67 structure. The resulting Ni@ZIF-67 exhibited excellent adsorption efficiency for removing MO from water. The adsorption performance of Ni-doped ZIF-67 was evaluated under different pH levels, contact times, and dye concentrations. The results showed that Ni@ZIF-67 adsorbed more dye in mildly acidic conditions (qe = 24.24 mg g−1 at pH 6) compared to acidic (qe = 17.69 mg g−1 at pH 2) and basic conditions (qe = 15.74 mg g−1 at pH 10). The adsorption data fit best with the Langmuir isotherm model, indicating a monolayer adsorption process. The maximum adsorption capacity achieved was 151.74 mg g−1, with excellent recyclability up to the fifth cycle. Additionally, within a 180 minute contact time, ZIF-67 and Ni-doped ZIF-67 nanocomposites adsorbed 68.5% and 82.9% of MO, respectively.189
4.2.2 Congo red (CR). CR is used in industries such as solar cells, pharmaceuticals, textiles, plastics, and papermaking. CR contains six aromatic rings and two azo functional groups, contributing to its toxicity. It can contaminate water, posing serious risks to humans and marine life, including plants and aquatic organisms. In humans, exposure to CR can lead to toxicity and health issues, such as mutations and respiratory problems. The contamination of water by CR is a significant environmental concern.190Abd El-Monaem et al.191 developed a bimetallic MOF composite film, Fe/ZnMOF-@CTS, for the removal of CR using an adsorption method. Remarkably, the Qmax of CR on Fe/MOF-5@CTS reached 219.78 mg g−1. In addition, the composite film retained 81.46% of its capacity after more than nine cycles. The selectivity tests revealed that the positively charged composite film exhibited higher selectivity for CR compared to C dyes. Based on practical experiments and analysis, the adsorption mechanism of CR on Fe/MOF-5@CTS is thought to involve electrostatic interactions, host–guest interactions, π–π interactions, and coordination bonds, as illustrated in Fig. 10.
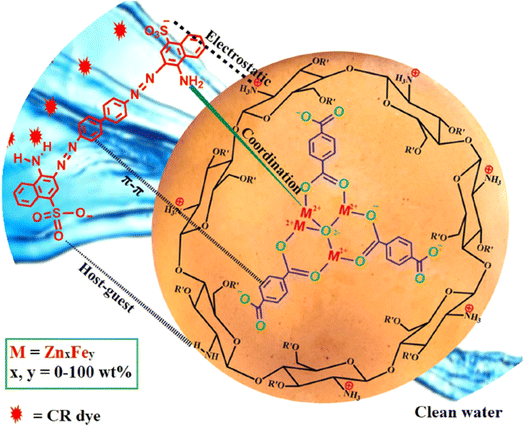 |
| Fig. 10 Possible mechanism for CR. From ref. 191 with permission. Copyright 2024, Springer. | |
Liu et al.192 synthesized a bimetallic CoFe-MOF and tested its effectiveness in removing CR from aqueous solutions, demonstrating its potential for treating wastewater containing organic dyes. The CoFe-MOF exhibited an impressive adsorption capacity of 1935.68 mg g−1 for CR, significantly higher than the capacities of monometallic Fe and Co MOFs, which were 775.19 mg g−1 and 628.93 mg g−1, respectively. This suggests that the CoFe-MOF has more defects, leading to enhanced adsorption efficiency. The results indicate that the synthesized MOF materials could be promising candidates for treating organic dye pollution, particularly A dyes, in wastewater.
Table 3 summarizes studies that utilized BMOFs for the removal of CR.
Table 3 Degradation of CR by BMOFs
Catalysts |
Method |
Catalyst dosage |
Initial concentration |
Temperature °C |
pH |
Performance% |
Ref. |
Lac@Co/Cu MOFs |
Adsorption |
10 mg |
100 mg L−1 |
40 |
7 |
95 |
193 |
Ni/Co MOFs |
Adsorption |
0.6 g L−1 |
0.4 g L−1 |
|
4 |
85 |
194 |
Fe/Al MOFs |
Adsorption |
0.167 mg mL−1 |
500 mg mL−1 |
25 |
5 |
96.7 |
195 |
ZIF Zn/Co |
Degradation |
20.0 mg |
5 mg L−1 |
— |
— |
100 |
196 |
Ni/Zn MOFs |
Adsorption |
20 mg |
200 mg L−1 |
30 |
3 |
— |
197 |
Co/Cu MOFs |
Degradation |
— |
100 mg L−1 |
50 |
3.5 |
95 |
198 |
Additionally, another type of dye is summarized in Table 4.
Table 4 Other anionic dye removal
Catalysts |
Dye |
Method |
Catalyst dosage |
Initial concentration |
Temperature °C |
pH |
Performance% |
Ref. |
Cu/Co MOFs |
Orange G |
Degradation |
50 mg L−1 |
0.2 mM |
25 |
Neutral |
96.4 |
199 |
NH2–MIL-101(Fe/Co) |
Orange G |
Degradation |
50 mg L−1 |
0.2 mM |
25 |
7 |
100 |
199 |
Fe/Ni MOFs |
MO |
Degradation |
— |
10 mg L−1 |
— |
7 |
99 |
200 |
Ag/Zn MOFs |
Reactive yellow 145 |
Degradation |
— |
0.4 g L−1 |
— |
— |
100 |
201 |
La/Ag-MOFs |
Sunset yellow |
Adsorption |
0.02 g |
0.02 g |
— |
4 |
— |
202 |
Cu/Sn MOFs |
Acid-blue 92 |
Degradation |
0.03 g |
7 × 10−5 M |
30 |
— |
86.9 |
203 |
La/Sn@MOF |
Tartrazine |
Adsorption |
0.02 g |
1.06 × 10−3 mmol L−1 |
25 |
6.41 |
98.3 |
204 |
Ag/Cu-MOFs PES |
Reactive black 5 and reactive red 120 |
Rejections |
— |
— |
— |
— |
96.4, 98.4 |
205 |
Cu/Zn ZIFs |
RG, RB and CR |
Degradation |
— |
50 mg L−1 |
— |
— |
68.3% |
206 |
Fe/Ni MIL-88 |
Eosin-Y |
Degradation |
5 mg |
20 mg |
— |
— |
— |
207 |
Cu/Fe MOFs |
Eosin-Y |
Adsorption |
0.005 g |
4 mg L−1 |
— |
— |
— |
208 |
Mn/Al MOFs |
EBT |
Degradation |
5.0 mg |
10 mg L−1 |
— |
— |
84.9–100 |
209 |
Fe/Ti MOFs |
Orange II |
Degradation |
100 mg L−1 |
50 mg L−1 |
25 |
5 |
100 |
210 |
Cu/Co ZIFs |
Acid orange II |
Degradation |
5 mg |
100 mg L−1 |
25 |
7 |
95.3 |
211 |
Zn/Co ZIFs |
Acid violet 7 |
Reduction |
0.2 g L−1 |
20 mg L−1 |
25 |
3.7 |
— |
212 |
GCN/M–FeBTC |
RR-195 |
Degradation |
0.5 g L−1 |
0.6 g L−1 |
— |
9 |
99.37 |
213 |
Ni/Co MOFs |
Reactive red |
Adsorption |
— |
100 mg L−1 |
25 |
— |
— |
214 |
4.3 Multiple dye removal
BMOFs feature two distinct metal ions within their structure, which can significantly enhance their properties compared to monometallic MOFs. These improvements often include greater stability, larger surface areas, and superior adsorption or catalytic activities. As a result, BMOFs are highly effective for a range of applications, including dye removal. Table 5 summarizes studies that utilized BMOFs for the removal of multiple dyes.
Table 5 Degradation of multiple dye by BMOFs
Catalysts |
Dyes |
Method |
Catalyst dosage |
Initial concentration |
pH |
Performance% |
Ref. |
Cu/Ni–BTC@SiO2 |
MO, MB |
Degradation |
10 mg L−1 |
20 mg L−1 |
7 |
98, 71 |
215 |
10 mg L−1 |
Zr/Cu MOFs |
RhB, MB |
Degradation |
— |
— |
7 |
96, 96 |
216 |
Co/Zn MOFs |
MB, MG RhB, MV-2B, CR |
Reduction |
20 mg |
0.04 mM, 0.0125, 0.0125, 0.038 and 0.02 mM |
— |
— |
217 |
Zn/Co MOFs |
MB, RR |
Adsorption |
— |
— |
— |
92, 91 |
217 |
Cu/Zr MOFs |
MO, MB |
Adsorption |
— |
— |
— |
— |
218 |
Fe/Ni MOFs |
MB, MO |
Adsorption |
10 mg |
20 mg L−1 |
6.5, 6.9 |
84.8 |
219 |
Ni/Zn MOFs |
MG, CR |
Adsorption |
0.25 g L−1 |
20 mg L−1 |
— |
— |
220 |
Fe/Cu MOFs |
MO, MB |
Reduction |
1 mg |
0.05 mM |
— |
52, 66 |
221 |
Co/Ni MOFs |
MB, CR, NR |
Adsorption |
— |
— |
5–10 |
98.34, 93.95, 94.42 |
222 |
Co/Fe MOFs |
MB, MO |
Adsorption |
0.01 g L−1 |
200 mg L−1 |
10, 4 |
70, 81 |
223 |
Co/Ni MOFs |
AB92, MO, and MB |
Adsorption |
— |
20 mg L−1 |
5 |
— |
224 |
Zn/Cu MOFs |
MG |
Degradation |
— |
10 mg L−1 |
4 |
89.7 |
225 |
5. Conclusions and prospects
A new material that functions as both an adsorbent and catalyst, with a high capacity for removing dyes, is eagerly anticipated. Recently, porous structured materials based on BMOFs have demonstrated potential in removing toxic dyes. This review focuses on the removal of both cationic (C) and anionic (A) dyes by various BMOFs. Based on the literature, it can be concluded that BMOFs can serve as superior adsorbents for removing A and C dyes from water compared to other nanomaterials, such as carbon dots (CDs), MOFs, metal oxides, and other materials, due to their higher surface area, pore geometries, ease of functionalization, and the presence of two metal nodes. BMOFs can also be useful in real-time applications.
The review highlights the importance of developing low-cost synthesis methods with minimal time requirements and suggests that more effort be put into real sample analysis using BMOFs. It stresses the need to consider the disposal of spent BMOFs and unremoved dyes after adsorption and degradation. Additionally, it outlines several challenges to be overcome, such as production and regeneration expenses, synthesis methods, stability, and practical real-time applications.
The potential of BMOFs as adsorbents or catalysts in water treatment is emphasized, with a recommendation for research focused on developing smart and straightforward BMOFs through green synthesis methods. Key areas of focus include large-scale production, achieving ultra-high surface area, increasing active sites, enhancing stability and selectivity, enabling ultra-fast removal rates, maximizing adsorption capacity, improving reusability, and facilitating real-time applications. The review suggests the possibility of synthesizing BMOFs using a simple, low-cost method and highlights the crucial role of environmental researchers in advancing research and development in this field.
Data availability
Data sharing is not applicable to this article as no datasets were generated or analysed during the current study.
Conflicts of interest
There are no conflicts to declare.
References
- C. Li, B. Xu, L. Chen, M. Jin, G. Yi and L. Chen, et al., Persulfate Activation by N-Doping Biochar from Peanut for Efficient Degradation of Phenol, Catal. Lett., 2023, 153(9), 2824–2837 CrossRef.
- M. S. Mustafa, N. N. Mohammad, F. H. Radha, K. F. Kayani, H. O. Ghareeb and S. J. Mohammed, Eco-friendly spectrophotometric methods for concurrent analysis of phenol, 2-aminophenol, and 4-aminophenol in ternary mixtures and water samples: assessment of environmental sustainability, RSC Adv., 2024, 14(23), 16045–16055 RSC.
- F. Lu and D. Astruc, Nanocatalysts and other nanomaterials for water remediation from organic pollutants, Coord. Chem. Rev., 2020, 408, 213180 CrossRef.
- S. H. Jang, Y. G. Jeong, B. G. Min, W. S. Lyoo and S. C. Lee, Preparation and lead ion removal property of hydroxyapatite/polyacrylamide composite hydrogels, J. Hazard. Mater., 2008, 159(2–3), 294–299 CrossRef PubMed.
- Y.-T. Zhou, H.-L. Nie, C. Branford-White, Z.-Y. He and L.-M. Zhu, Removal of Cu2+ from aqueous solution by chitosan-coated magnetic nanoparticles modified with α-ketoglutaric acid, J. Colloid Interface Sci., 2009, 330(1), 29–37 CrossRef PubMed.
- C. C. Okafor, C. N. Madu, C. C. Ajaero, J. C. Ibekwe, C. A. Nzekwe and C. Okafor, et al., Sustainable management of textile and clothing, Clean Technol. Recycl., 2021, 1, 70–87 Search PubMed.
- S. Li, Y. Cui, M. Wen and G. Ji, Toxic effects of methylene blue on the growth, reproduction and physiology of Daphnia magna, Toxics, 2023, 11(7), 594 CrossRef CAS PubMed.
- E. Baldev, D. MubarakAli, A. Ilavarasi, D. Pandiaraj, K. A. S. S. Ishack and N. Thajuddin, Degradation of synthetic dye, rhodamine B to environmentally non-toxic products using microalgae, Colloids Surf., B, 2013, 105, 207–214 CrossRef CAS PubMed.
- M. F. Hanafi and N. Sapawe, A review on the water problem associate with organic pollutants derived from phenol, methyl orange, and Remazol brilliant blue dyes, Mater. Today: Proc., 2020, 31, A141–A150 Search PubMed.
- S. I. Siddiqui, E. S. Allehyani, S. A. Al-Harbi, Z. Hasan, M. A. Abomuti and H. K. Rajor, et al., Investigation of Congo red toxicity towards different living organisms: a review, Processes, 2023, 11(3), 807 CrossRef CAS.
- L. Ayed, A. Mahdhi, A. Cheref and A. Bakhrouf, Decolorization and degradation of azo dye methyl red by an isolated Sphingomonas paucimobilis: biotoxicity and metabolites characterization, Desalination, 2011, 274(1–3), 272–277 CrossRef CAS.
- H. B. Slama, A. C. Bouket, Z. Pourhassan, F. N. Alenezi, A. Silini and H. Cherif-Silini, et al., Diversity of synthetic dyes from textile industries, discharge impacts and treatment methods, Appl. Sci., 2021, 11(14), 6255 CrossRef CAS.
- A. Paz, J. Carballo, M. J. Pérez and J. M. Domínguez, Biological treatment of model dyes and textile wastewaters, Chemosphere, 2017, 181, 168–177 CrossRef CAS.
- F. Vazquez-Ortega, I. Lagunes and A. Trigos, Cosmetic dyes as potential photosensitizers of singlet oxygen generation, Dyes Pigm., 2020, 176, 108248 CrossRef CAS.
- S. Mandal and J. Venkatramani, A review of plant-based natural dyes in leather application with a special focus on color fastness characteristics, Environ. Sci. Pollut. Res., 2023, 30(17), 48769–48777 CrossRef CAS PubMed.
- A. Durazzo, M. Carocho, S. Heleno, L. Barros, E. B. Souto and A. Santini, et al., Food dyes and health: Literature quantitative research analysis, Measurement: Food, 2022, 7, 100050 Search PubMed.
- A. Gičević, L. Hindija and A. Karačić, Toxicity of azo dyes in pharmaceutical industry, in CMBEBIH 2019: Proceedings of the International Conference on Medical and Biological Engineering, 16–18 May 2019, Banja
Luka, Bosnia and Herzegovina, Springer, 2020, pp. 581–587 Search PubMed.
- D. Yadav and J. Dutta, Advancing environmental sustainability: recent trends and developments in treatment methods for paint industry wastewater, J. Water Process Eng., 2024, 61, 105290 CrossRef.
- S. Nair, B. Manu and A. Azhoni, Sustainable treatment of paint industry wastewater: current techniques and challenges, J. Environ. Manage., 2021, 296, 113105 CrossRef PubMed.
- M. Yadav and H. S. Yadav, Applications of ligninolytic enzymes to pollutants, wastewater, dyes, soil, coal, paper and polymers, Environ. Chem. Lett., 2015, 13, 309–318 CrossRef CAS.
- C. Ram, P. Rani, K. A. Gebru and M. G. M. Abrha, Pulp and paper industry wastewater treatment: use of microbes and their enzymes, Phys. Sci. Rev., 2020, 5(10), 20190050 Search PubMed.
- P. Singh, N. Rani, S. Kumar, P. Kumar, B. Mohan and V. Bhankar, et al., Assessing the biomass-based carbon dots and their composites for photocatalytic treatment of wastewater, J. Clean. Prod., 2023, 413, 137474 CrossRef CAS.
- P. O. Oladoye, T. O. Ajiboye, W. C. Wanyonyi, E. O. Omotola and M. E. Oladipo, Ozonation, electrochemical, and biological methods for the remediation of malachite green dye wastewaters: a mini review, Sustainable Chemistry for the Environment, 2023, 100033 CrossRef.
- U. Chadha, S. K. Selvaraj, S. V. Thanu, V. Cholapadath, A. M. Abraham and M. Manoharan, et al., A review of the function of using carbon nanomaterials in membrane filtration for contaminant removal from wastewater, Mater. Res. Express, 2022, 9(1), 12003 CAS.
- A. M. Elgarahy, K. Z. Elwakeel, S. H. Mohammad and G. A. Elshoubaky, A critical review of biosorption of dyes, heavy metals and metalloids from wastewater as an efficient and green process, Clean. Eng. Technol., 2021, 4, 100209 CrossRef.
- S. Mishra, L. Cheng and A. Maiti, The utilization of agro-biomass/byproducts for effective bio-removal of dyes from dyeing wastewater: a comprehensive review, J. Environ. Chem. Eng., 2021, 9(1), 104901 CrossRef CAS.
- A. H. Jagaba, I. Abdulazeez, D. U. Lawal, A. C. Affam, N. D. Mu'azu and U. B. Soja, et al., A review on the application of biochar as an innovative and sustainable biocarrier material in moving bed biofilm reactors for dye removal from environmental matrices, Environ. Geochem. Health, 2024, 46(9), 1–32 Search PubMed.
- S. Murshid, A. Antonysamy, G. Dhakshinamoorthy, A. Jayaseelan and A. Pugazhendhi, A review on biofilm-based reactors for wastewater treatment: recent advancements in biofilm carriers, kinetics, reactors, economics, and future perspectives, Sci. Total Environ., 2023, 892, 164796 CrossRef CAS PubMed.
- Z. Al-Qodah, M. Tawalbeh, M. Al-Shannag, Z. Al-Anber and K. Bani-Melhem, Combined electrocoagulation processes as a novel approach for enhanced pollutants removal: a state-of-the-art review, Sci. Total Environ., 2020, 744, 140806 CrossRef CAS PubMed.
- H. Zhu, S. Chen and Y. Luo, Adsorption mechanisms of hydrogels for heavy metal and organic dyes removal: a short review, J. Agric. Food Res., 2023, 12, 100552 CAS.
- H. M. Solayman, M. A. Hossen, A. A. Aziz, N. Y. Yahya, K. H. Leong and L. C. Sim, et al., Performance evaluation of dye wastewater treatment technologies: a review, J. Environ. Chem. Eng., 2023, 11(3), 109610 CrossRef CAS.
- M. Harja, G. Buema and D. Bucur, Recent advances in removal of Congo red dye by adsorption using an industrial waste, Sci. Rep., 2022, 12(1), 6087 CrossRef CAS PubMed.
- S. Sağlam, F. N. Türk and H. Arslanoğlu, Use and applications of metal-organic frameworks (MOF) in dye adsorption, J. Environ. Chem. Eng., 2023, 110568 CrossRef.
- M. B. Wazir, M. Daud, F. Ali and M. A. Al-Harthi, Dendrimer assisted dye-removal: a critical review of adsorption and catalytic degradation for wastewater treatment, J. Mol. Liq., 2020, 315, 113775 CrossRef.
- R. Begum, J. Najeeb, A. Sattar, K. Naseem, A. Irfan and A. G. Al-Sehemi, et al., Chemical reduction of methylene blue in the presence of nanocatalysts: a critical review, Rev. Chem. Eng., 2020, 36(6), 749–770 CrossRef.
- A. Rafiq, M. Ikram, S. Ali, F. Niaz, M. Khan and Q. Khan, et al., Photocatalytic degradation of dyes using semiconductor photocatalysts to clean industrial water pollution, J. Ind. Eng. Chem., 2021, 97, 111–128 CrossRef.
- S. Marimuthu, A. J. Antonisamy, S. Malayandi, K. Rajendran, P.-C. Tsai and A. Pugazhendhi, et al., Silver nanoparticles in dye effluent treatment: a review on synthesis, treatment methods, mechanisms, photocatalytic degradation, toxic effects and mitigation of toxicity, J. Photochem. Photobiol., B, 2020, 205, 111823 CrossRef PubMed.
- A. N. M. A. Haque, N. Sultana, A. S. M. Sayem and S. A. Smriti, Sustainable adsorbents from plant-derived agricultural wastes for anionic dye removal: a review, Sustainability, 2022, 14(17), 11098 CrossRef.
- G. A. Ismail and H. Sakai, Review on effect of different type of dyes on advanced oxidation processes (AOPs) for textile color removal, Chemosphere, 2022, 291, 132906 CrossRef CAS PubMed.
- D. A. Kader, D. M. Aziz, S. J. Mohammed, N. N. N. Maarof, W. O. Karim and S. A. Mhamad, et al., Green synthesis of ZnO/catechin nanocomposite: comprehensive characterization, optical study, computational analysis, biological applications and molecular docking, Mater. Chem. Phys., 2024, 319, 129408 CrossRef CAS.
- N. M. Hosny, I. Gomaa and M. G. Elmahgary, Adsorption of polluted dyes from water by transition metal oxides: a review, Appl. Surf. Sci. Adv., 2023, 15, 100395 CrossRef.
- S. Yadav, K. Shakya, A. Gupta, D. Singh, A. R. Chandran and A. V. Aanappalli, et al., A review on degradation of organic dyes by using metal oxide semiconductors, Environ. Sci. Pollut. Res., 2023, 30(28), 71912–71932 CrossRef CAS PubMed.
- H. R. Ahmed, M. A. Salih, N. N. M. Agha, D. I. Tofiq, M. A. H. Karim and K. F. Kayani, et al., Enhanced methyl orange removal in aqueous solutions using bio-catalytic metal oxides derived from pomegranate peel waste: a green chemistry evaluation, React. Kinet. Mech. Catal., 2024, 1–24 Search PubMed.
- R. M. S. Sendão, J. C. G. E. da Silva and L. P. da Silva, Applications of Fluorescent Carbon Dots as Photocatalysts: A Review, Catalysts, 2023, 13(1), 179 CrossRef.
- A. G. El-Shamy, An efficient removal of methylene blue dye by adsorption onto carbon dot@zinc peroxide embedded poly vinyl alcohol (PVA/CZnO2) nano-composite: a novel reusable adsorbent, Polymer, 2020, 202, 122565 CrossRef CAS.
- K. F. Kayani, S. J. Mohammed, D. Ghafoor, M. K. Rahim and H. R. Ahmed, Carbon dot as fluorescence sensor for glutathione in human serum samples: a review, Mater. Adv., 2024, 5(11), 4618–4633 RSC.
- K. F. Kayani, S. J. Mohammed, N. N. Mohammad, G. H. Abdullah, D. A. Kader and N. S. H. Mustafa, Ratiometric fluorescence detection of tetracycline in milk and tap water with smartphone assistance for visual pH sensing using innovative dual-emissive phosphorus-doped carbon dots, Food Control, 2024, 164, 110611 CrossRef CAS.
- K. F. Kayani, M. K. Rahim, S. J. Mohammed, H. R. Ahmed, M. S. Mustafa and S. B. Aziz, Recent Progress in Folic Acid Detection Based on Fluorescent Carbon Dots as Sensors: A Review, J. Fluoresc., 2024 DOI:10.1007/s10895-024-03728-3.
- K. F. Kayani and C. N. Abdullah, A Dual-Mode Detection Sensor Based on Nitrogen-Doped Carbon Dots for Visual Detection of Fe(III) and Ascorbic Acid via a Smartphone, J. Fluoresc., 2024 DOI:10.1007/s10895-024-03604-0.
- K. F. Kayani, O. B. A. Shatery, M. S. Mustafa, A. H. Alshatteri, S. J. Mohammed and S. B. Aziz, Environmentally sustainable synthesis of whey-based carbon dots for ferric ion detection in human serum and water samples: evaluating the greenness of the method, RSC Adv., 2024, 14(8), 5012–5021 RSC.
- S. J. Mohammed, F. E. Hawaiz, S. B. Aziz and S. H. Al-Jaf, Organic soluble nitrogen-doped carbon dots (ONCDs)
to reduce the optical band gap of PVC polymer: breakthrough in polymer composites with improved optical properties, Opt. Mater., 2024, 149, 115014 CrossRef CAS.
- K. F. Kayani, S. J. Mohammed, N. N. Mohammad, A. M. Abdullah, D. I. Tofiq and M. S. Mustafa, et al., Sulfur quantum dots for fluorescence sensing in biological and pharmaceutical samples: a review, Mater. Adv., 2024, 5, 6351–6367 RSC.
- S. Soni, P. K. Bajpai, J. Mittal and C. Arora, Utilisation of cobalt doped Iron based MOF for enhanced removal and recovery of methylene blue dye from waste water, J. Mol. Liq., 2020, 314, 113642 CrossRef CAS.
- R. Yang, Q. Peng, B. Yu, Y. Shen and H. Cong, Yolk-shell Fe3O4@ MOF-5 nanocomposites as a heterogeneous Fenton-like catalyst for organic dye removal, Sep. Purif. Technol., 2021, 267, 118620 CrossRef CAS.
- M. Firoozi, Z. Rafiee and K. Dashtian, New MOF/COF hybrid as a robust adsorbent for simultaneous removal of auramine O and rhodamine B dyes, ACS Omega, 2020, 5(16), 9420–9428 CrossRef CAS.
- M. S. Khan, Y. Li, D.-S. Li, J. Qiu, X. Xu and H. Y. Yang, A review of metal-organic framework (MOF) materials as an effective photocatalyst for degradation of organic pollutants, Nanoscale Adv., 2023, 5, 6318–6348 RSC.
- A. Das and A. K. Das, A functionalized Hf (iv)–organic framework introducing an efficient, recyclable, and size-selective heterogeneous catalyst for MPV reduction, New J. Chem., 2023, 47(11), 5347–5355 RSC.
- Z. Yang, Y.-W. Chen, Y.-F. Jin, Z. Jin, H.-S. Xie and X.-S. Cong, et al., Ni, Co-embedded MOF-derived N-doped bimetallic porous carbon for adsorption–photocatalytic degradation of organic dyes and antibiotics, ACS Omega, 2024, 9(10), 11356–11365 CrossRef CAS PubMed.
- A. Tiwari, P. S. Sagara, V. Varma and J. K. Randhawa, Bimetallic metal organic frameworks as magnetically separable heterogeneous catalysts and photocatalytic dye degradation, ChemPlusChem, 2019, 84(1), 136–141 CrossRef CAS PubMed.
- A. Das, D. Chavda, M. Manna and A. K. Das, Dual Active Hf(IV)-Organic Framework Acting Smart Sensor for Aqueous Phase Detection of FOX-7 and Recyclable Efficient Heterogeneous Catalyst on Knoevenagel Condensation, New J. Chem., 2024 10.1039/D4NJ02344G.
- M. J. Uddin, R. E. Ampiaw and W. Lee, Adsorptive removal of dyes from wastewater using a metal-organic framework: a review, Chemosphere, 2021, 284, 131314 CrossRef CAS PubMed.
- K. F. Kayani and K. M. Omer, A red luminescent europium metal organic framework (Eu-MOF) integrated with a paper strip using smartphone visual detection for determination of folic acid in pharmaceutical formulations, New J. Chem., 2022, 46(17), 8152–8161 RSC.
- K. F. Kayani, N. N. Mohammad, D. A. Kader, S. J. Mohammed, D. A. Shukur and A. H. Alshatteri, et al., Ratiometric Lanthanide Metal-Organic Frameworks (MOFs) for Smartphone-Assisted Visual Detection of Food Contaminants and Water: A Review, ChemistrySelect, 2023, 8(47), e202303472 CrossRef.
- O. B. A. Shatery, K. F. Kayani, M. S. Mustafa and S. J. Mohammed, Rational design for enhancing sensitivity and robustness of a probe via encapsulation of carbon dots into a zeolitic imidazolate framework-8 for quantification of tetracycline in milk with greenness evaluation, Res. Chem. Intermed., 2024 DOI:10.1007/s11164-024-05271-z.
- Z. Gan, X. Hu, X. Xu, W. Zhang, X. Zou and J. Shi, et al., A portable test strip based on fluorescent europium-based metal–organic framework for rapid and visual detection of tetracycline in food samples, Food Chem., 2021, 354(March), 129501 CrossRef PubMed.
- J. Xiong, L. Yang, L. X. Gao, P. P. Zhu, Q. Chen and K. J. Tan, A highly fluorescent lanthanide metal-organic framework as dual-mode visual sensor for berberine hydrochloride and tetracycline, Anal. Bioanal. Chem., 2019, 411(23), 5963–5973 CrossRef PubMed.
- T. Friić, New opportunities for materials synthesis using mechanochemistry, J. Mater. Chem., 2010, 20(36), 7599–7605 RSC.
- A. H. Chughtai, N. Ahmad, H. A. Younus, A. Laypkov and F. Verpoort, Metal-organic frameworks: versatile heterogeneous catalysts for efficient catalytic organic transformations, Chem. Soc. Rev., 2015, 44(19), 6804–6849 RSC.
- D. Feng, Z. Y. Gu, J. R. Li, H. L. Jiang, Z. Wei and H. C. Zhou, Zirconium-metalloporphyrin PCN-222: mesoporous metal-organic frameworks with ultrahigh stability as biomimetic catalysts, Angew. Chem., Int. Ed., 2012, 51(41), 10307–10310 CrossRef PubMed.
- H. Li, S. Xu, J. Du, J. Tang and Q. Zhou, Cu@ Co-MOFs as a novel catalyst of peroxymonosulfate for the efficient removal of methylene blue, RSC Adv., 2019, 9(17), 9410–9420 RSC.
- J. Chen, Q. Zhang, F. Xu and S. Li, Bimetallic organic frame nanosheet fluorescent probe used for detecting tetracycline and folic acid, Microchem. J., 2021, 170(July), 106673 CrossRef CAS.
- H. D. Lawson, S. P. Walton and C. Chan, Metal-Organic Frameworks for Drug Delivery: A Design Perspective, ACS Appl. Mater. Interfaces, 2021, 13(6), 7004–7020 CrossRef CAS PubMed.
- X. Chen, R. Tong, Z. Shi, B. Yang, H. Liu and S. Ding, et al., MOF Nanoparticles with Encapsulated Autophagy Inhibitor in Controlled Drug Delivery System for Antitumor, ACS Appl. Mater. Interfaces, 2018, 10(3), 2328–2337 CrossRef CAS PubMed.
- H. Zhang, X. Shi, J. Li, P. Kumar and B. Liu, Selective dye adsorption by zeolitic imidazolate framework-8 loaded UiO-66-NH2, Nanomaterials, 2019, 9(9), 1283 CrossRef CAS.
- M. Yang and Q. Bai, Flower-like hierarchical Ni-Zn MOF microspheres: efficient adsorbents for dye removal, Colloids Surf., A, 2019, 582(August), 123795, DOI:10.1016/j.colsurfa.2019.123795.
- V. F. Yusuf, N. I. Malek and S. K. Kailasa, Review on Metal-Organic Framework Classification, Synthetic Approaches, and Influencing Factors: Applications in Energy, Drug Delivery, and Wastewater Treatment, ACS Omega, 2022, 7(49), 44507–44531 CrossRef CAS PubMed.
- S.-H. Guo, X.-J. Qi, H.-M. Zhou, J. Zhou, X.-H. Wang and M. Dong, et al., A bimetallic-MOF catalyst for efficient CO2 photoreduction from simulated flue gas to value-added formate, J. Mater. Chem. A, 2020, 8(23), 11712–11718 RSC.
- R.-B. Lin, S. Xiang, W. Zhou and B. Chen, Microporous metal-organic framework materials for gas separation, Chem, 2020, 6(2), 337–363 CAS.
- D. Yuan, C. Shang, J. Cui, W. Zhang and Y. Kou, Removal of Cr (VI) from aqueous solutions via simultaneous reduction and adsorption by modified bimetallic MOF-derived carbon material Cu@ MIL-53 (Fe): performance, kinetics, and mechanism, Environ. Res., 2023, 216, 114616 CrossRef CAS PubMed.
- X. Du, S. Feng, J. Luo, Y. Zhuang, W. Song and X. Li, et al., Pebax mixed matrix membrane with bimetallic CeZr-MOFs to enhance CO2 separation, Sep. Purif. Technol., 2023, 322, 124251 CrossRef CAS.
- A. Kumari, S. Kaushal and P. P. Singh, Bimetallic metal organic frameworks heterogeneous catalysts: design, construction, and applications, Mater. Today Energy, 2021, 20, 100667 CrossRef CAS.
- P. Li, Y. Bai, G. Zhang, X. Guo, X. Meng and H. Pang, Surface-halogen-introduced 2D NiCo bimetallic MOFs via a modulation method for elevated electrochemical glucose sensing, Inorg. Chem. Front., 2022, 9(22), 5853–5861 RSC.
- K. F. Kayani and A. M. Abdullah, Eco-Friendly Fluorescent Detection Method for Cu2+ ions Combined with Smartphone-Integrated Paper Strip Sensors Based on Highly Fluorescent 2-Aminoterephthalic Acid in Milk Samples, J. Food Compos. Anal., 2024, 106577 CrossRef CAS.
- C. Rejeeth, A. Sharma, R. S. Kumar, A. I. Almansour, N. Arumugam and N. B. Varukattu, et al., Bimetallic Metal–Organic Framework Nanoparticles for Monitoring Metabolic Changes in Cardiovascular Disorders, ACS Appl. Nano Mater., 2023, 6(9), 8071–8081 CrossRef CAS.
- S. Sanati, R. Abazari, J. Albero, A. Morsali, H. García and Z. Liang, et al., Metal–organic framework derived bimetallic materials for electrochemical energy storage, Angew. Chem., Int. Ed., 2021, 60(20), 11048–11067 CrossRef CAS PubMed.
- A. Kausar, S. T. Zohra, S. Ijaz, M. Iqbal, J. Iqbal and I. Bibi, et al., Cellulose-based materials and their adsorptive removal efficiency for dyes: a review, Int. J. Biol. Macromol., 2023, 224, 1337–1355 CrossRef CAS PubMed.
- A. C. Sadiq, A. Olasupo, W. S. W. Ngah, N. Y. Rahim and F. B. M. Suah, A decade development in the application of chitosan-based materials for dye adsorption: a short review, Int. J. Biol. Macromol., 2021, 191, 1151–1163 CrossRef CAS PubMed.
- J. Wang, J. Zhang, L. Han, J. Wang, L. Zhu and H. Zeng, Graphene-based materials for adsorptive removal of pollutants from water and underlying interaction mechanism, Adv. Colloid Interface Sci., 2021, 289, 102360 CrossRef CAS PubMed.
- Z. Cigeroglu, N. El Messaoudi, Z. M. Şenol, G. Baskan, J. Georgin and S. Gubernat, Clay-based nanomaterials and their adsorptive removal efficiency for dyes and antibiotics: a review, Mater. Today Sustain., 2024, 100735 Search PubMed.
- M. Adel, M. A. Ahmed, M. A. Elabiad and A. A. Mohamed, Removal of heavy metals and dyes from wastewater using graphene oxide-based nanomaterials: a critical review, Environ. Nanotechnol., Monit. Manage., 2022, 18, 100719 CAS.
- Y.-G. Park, S.-N. Nam, M. Jang, C. M. Park, N. Her and J. Sohn, et al., Boron nitride-based nanomaterials as adsorbents in water: a review, Sep. Purif. Technol., 2022, 288, 120637 CrossRef CAS.
- V. K. Gupta, Application of low-cost adsorbents for dye removal–a review, J. Environ. Manage., 2009, 90(8), 2313–2342 CrossRef CAS PubMed.
- K. Hunger, Industrial Dyes: Chemistry, Properties, Applications, John Wiley & Sons, 2007 Search PubMed.
- Y. Zhou, J. Lu, Y. Zhou and Y. Liu, Recent advances for dyes removal using novel adsorbents: a review, Environ. Pollut., 2019, 252, 352–365 CrossRef CAS PubMed.
- G. Ye, H. Wang, X. Zeng, L. Wang and J. Wang, Defect-rich bimetallic UiO-66 (Hf-Zr): solvent-free rapid synthesis and robust ambient-temperature oxidative desulfurization performance, Appl. Catal., B, 2021, 299, 120659 CrossRef CAS.
- M. F. Sanad, A. R. P. Santiago, S. A. Tolba, M. A. Ahsan, O. Fernandez-Delgado and M. S. Adly, et al., Co–Cu bimetallic metal organic framework catalyst outperforms the Pt/C benchmark for oxygen reduction, J. Am. Chem. Soc., 2021, 143(10), 4064–4073 CrossRef PubMed.
- Q. Liu, H. Cong and H. Deng, Deciphering the spatial arrangement of metals and correlation to reactivity in multivariate metal–organic frameworks, J. Am. Chem. Soc., 2016, 138(42), 13822–13825 CrossRef PubMed.
- S. Li, Y. Gao, N. Li, L. Ge, X. Bu and P. Feng, Transition metal-based bimetallic MOFs and MOF-derived catalysts for electrochemical oxygen evolution reaction, Energy Environ. Sci., 2021, 14(4), 1897–1927 RSC.
- J. Luo, X. Luo, Y. Gan, X. Xu, B. Xu and Z. Liu, et al., Advantages of bimetallic organic frameworks in the adsorption, catalysis and detection for water contaminants, Nanomaterials, 2023, 13(15), 2194 CrossRef PubMed.
- X. Yang and Q. Xu, Bimetallic metal–organic frameworks for gas storage and separation, Cryst. Growth Des., 2017, 17(4), 1450–1455 CrossRef.
- I. Soni, P. Kumar and G. K. Jayaprakash, Recent advancements in the synthesis and electrocatalytic activity of two-dimensional metal–organic framework with bimetallic nodes for energy-related applications, Coord. Chem. Rev., 2022, 472, 214782 CrossRef.
- Y. Liu, X. Liu, L. Feng, L. Shao, S. Li and J. Tang, et al., Two-dimensional metal–organic framework nanosheets: synthesis and applications in electrocatalysis and photocatalysis, ChemSusChem, 2022, 15(10), e202102603 CrossRef CAS PubMed.
- S. Liu, Y. Qiu, Y. Liu, W. Zhang, Z. Dai and D. Srivastava, et al., Recent advances in bimetallic metal–organic frameworks (BMOFs): synthesis, applications and challenges, New J. Chem., 2022, 46(29), 13818–13837 RSC.
- D. Jiang, Q. Zeng, Z. Wang, L. Yi, Y. Zhou and J. Zhu, Synthesis of the coordination microenvironment of HMOFs by heterogeneous metals and their remediation applications in the environmental field, Coord. Chem. Rev., 2024, 519, 216099 CrossRef CAS.
- N. Raza, T. Kumar, V. Singh and K.-H. Kim, Recent advances in bimetallic metal-organic framework as a potential candidate for supercapacitor electrode material, Coord. Chem. Rev., 2021, 430, 213660 CrossRef CAS.
- I. Saini, V. Singh and S. Hamad, Recent development in bimetallic metal organic frameworks as photocatalytic material, Inorg. Chem. Commun., 2023, 111897 Search PubMed.
- M. Y. Masoomi, A. Morsali, A. Dhakshinamoorthy and H. Garcia, Mixed-Metal MOFs: Unique Opportunities in Metal–Organic Framework (MOF) Functionality and Design, Angew. Chem., 2019, 131(43), 15330–15347 CrossRef.
- L. Chen, H.-F. Wang, C. Li and Q. Xu, Bimetallic metal–organic frameworks and their derivatives, Chem. Sci., 2020, 11(21), 5369–5403 RSC.
- K. R. Chithra, S. M. Rao, M. V. Varsha and G. Nageswaran, Bimetallic Metal-Organic Frameworks (BMOF) and BMOF-Incorporated Membranes for Energy and Environmental Applications, ChemPlusChem, 2023, 88(3), e202200420 CrossRef CAS PubMed.
- R. K. Pandey, Bimetallic metal–organic frameworks (BMOFs) and their potential applications, in Metal–Organic Frameworks for Carbon Capture and Energy, ACS Publications, 2021, pp. 3–15 Search PubMed.
- W. Chen, L. Du and C. Wu, Hydrothermal synthesis of MOFs, in Metal–Organic Frameworks for Biomedical Applications, Elsevier, 2020, pp. 141–157 Search PubMed.
- C. Mckinstry, R. J. Cathcart, E. J. Cussen, A. J. Fletcher, S. V. Patwardhan and J. Sefcik, Scalable continuous solvothermal synthesis of metal organic framework (MOF-5) crystals, Chem. Eng. J., 2016, 285, 718–725 CrossRef CAS.
- X. Wu, Z. Bao, B. Yuan, J. Wang, Y. Sun and H. Luo, et al., Microwave synthesis and characterization of MOF-74 (M= Ni, Mg) for gas separation, Microporous Mesoporous Mater., 2013, 180, 114–122 CrossRef CAS.
- O. J. de Lima Neto, A. C. de Oliveira Frós, B. S. Barros, A. F. de Farias Monteiro and J. Kulesza, Rapid and efficient electrochemical synthesis of a zinc-based nano-MOF for Ibuprofen adsorption, New J. Chem., 2019, 43(14), 5518–5524 RSC.
- K. Yu, Y. R. Lee, J. Y. Seo, K. Y. Baek, Y. M. Chung and W. S. Ahn, Sonochemical synthesis of Zr-based porphyrinic MOF-525 and MOF-545: enhancement in catalytic and adsorption properties, Microporous Mesoporous Mater., 2021, 316, 110985 CrossRef CAS.
- G. Cai, X. Ma, M. Kassymova, K. Sun, M. Ding and H.-L. Jiang, Large-scale production of hierarchically porous metal–organic frameworks by a reflux-assisted post-synthetic ligand substitution strategy, ACS Cent. Sci., 2021, 7(8), 1434–1440 CrossRef CAS PubMed.
- M. Y. Masoomi, A. Morsali, A. Dhakshinamoorthy and H. Garcia, Mixed-metal MOFs: unique opportunities in metal–organic framework (MOF) functionality and design, Angew. Chem., 2019, 131(43), 15330–15347 CrossRef.
- A. Nowacka, P. Briantais, C. Prestipino and F. X. Llabres i Xamena, Facile “green” aqueous synthesis of mono-and bimetallic trimesate metal–organic frameworks, Cryst. Growth Des., 2019, 19(9), 4981–4989 CrossRef CAS.
- S. Sanati, A. Morsali and H. Garcia, First-row transition metal-based materials derived from bimetallic metal–organic frameworks as highly efficient electrocatalysts for electrochemical water splitting, Energy Environ. Sci., 2022, 15(8), 3119–3151 RSC.
- A. F. Sapnik, H. S. Geddes, E. M. Reynolds, H. H.-M. Yeung and A. L. Goodwin, Compositional inhomogeneity and tuneable thermal expansion in mixed-metal ZIF-8 analogues, Chem. Commun., 2018, 54(69), 9651–9654 RSC.
- A. D. Burrows, Mixed-component metal–organic frameworks (MC-MOFs): enhancing functionality through solid solution formation and surface modifications, CrystEngComm, 2011, 13(11), 3623–3642 RSC.
- R. Abazari, A. R. Amani-Ghadim, A. M. Z. Slawin, C. L. Carpenter-Warren and A. M. Kirillov, Non-calcined layer-pillared Mn0.5Zn0.5 bimetallic–organic framework as a promising electrocatalyst for oxygen evolution reaction, Inorg. Chem., 2022, 61(25), 9514–9522 CrossRef CAS PubMed.
- M. Lammert, C. Glißmann and N. Stock, Tuning the stability of bimetallic Ce (IV)/Zr (IV)-based MOFs with UiO-66 and MOF-808 structures, Dalton Trans., 2017, 46(8), 2425–2429 RSC.
- R. Zou, P. Li, Y. Zeng, J. Liu, R. Zhao and H. Duan, et al., Bimetallic Metal-Organic Frameworks: Probing the Lewis Acid Site for CO2 Conversion, Small, 2016, 12(17), 2334–2343 CrossRef CAS PubMed.
- R. Wang, B.-T. Liu, S. Zhang, X.-S. Huang, L. Xie and T.-F. Liu, et al., Design of a Metal-Organic Framework with Preset Binding Sites for Crystallographic Visualized Metalation, Small Struct., 2022, 3(10), 2200148 CrossRef CAS.
- Y. Liu, G. Qiu, A. Yan, Y. Liu, Y. Niu and R. Qu, et al., Preparation of metal organic framework materials with defects via a mixed-metallic centers strategy for enhanced removal of organic dye, J. Mol. Liq., 2023, 370, 121016 CrossRef CAS.
- L. Chen, B. Huang, X. Qiu, X. Wang, R. Luque and Y. Li, Seed-mediated growth of MOF-encapsulated Pd@ Ag core–shell nanoparticles: toward advanced room temperature nanocatalysts, Chem. Sci., 2016, 7(1), 228–233 RSC.
- V. K. Gupta, R. Kumar, A. Nayak, T. A. Saleh and M. A. Barakat, Adsorptive removal of dyes from aqueous solution onto carbon nanotubes: a review, Adv. Colloid Interface Sci., 2013, 193, 24–34 CrossRef PubMed.
- A. M. Ghaedi and A. Vafaei, Applications of artificial neural networks for adsorption removal of dyes from aqueous solution: a review, Adv. Colloid Interface Sci., 2017, 245, 20–39 CrossRef PubMed.
- A. Kausar, M. Iqbal, A. Javed, K. Aftab, H. N. Bhatti and S. Nouren, Dyes adsorption using clay and modified clay: a review, J. Mol. Liq., 2018, 256, 395–407 CrossRef.
- G. Sriram, M. Kigga, U. T. Uthappa, R. M. Rego, V. Thendral and T. Kumeria, et al., Naturally available diatomite and their surface modification for the removal of hazardous dye and metal ions: a review, Adv. Colloid Interface Sci., 2020, 282, 102198 CrossRef PubMed.
- R. E. Abouzeid, R. Khiari, N. El-Wakil and A. Dufresne, Current state and new trends in the use of cellulose nanomaterials for wastewater treatment, Biomacromolecules, 2018, 20(2), 573–597 CrossRef PubMed.
- H. Sadegh, G. A. M. Ali, V. K. Gupta, A. S. H. Makhlouf, R. Shahryari-Ghoshekandi and M. N. Nadagouda, et al., The role of nanomaterials as effective adsorbents and their applications in wastewater treatment, J. Nanostruct. Chem., 2017, 7, 1–14 CrossRef.
- P. Sharma, H. Kaur, M. Sharma and V. Sahore, A review on applicability of naturally available adsorbents for the removal of hazardous dyes from aqueous waste, Environ. Monit. Assess., 2011, 183, 151–195 CrossRef CAS PubMed.
- P. N. E. Diagboya and E. D. Dikio, Silica-based mesoporous materials; emerging designer adsorbents for aqueous pollutants removal and water treatment, Microporous Mesoporous Mater., 2018, 266, 252–267 CrossRef CAS.
- D. Karadag, E. Akgul, S. Tok, F. Erturk, M. A. Kaya and M. Turan, Basic and reactive dye removal using natural and modified zeolites, J. Chem. Eng. Data, 2007, 52(6), 2436–2441 CrossRef CAS.
- M. A. M. Salleh, D. K. Mahmoud, W. A. W. A. Karim and A. Idris, Cationic and anionic dye adsorption by agricultural solid wastes: a comprehensive review, Desalination, 2011, 280(1–3), 1–13 Search PubMed.
- M. Khodaie, N. Ghasemi, B. Moradi and M. Rahimi, Removal of methylene blue from wastewater by adsorption onto ZnCl2 activated corn husk carbon equilibrium studies, J. Chem., 2013, 2013(1), 383985 CrossRef.
- A. S. Eltaweil, E. M. A. El-Monaem, G. M. El-Subruiti, M. M. A. El-Latif and A. M. Omer, Fabrication of UiO-66/MIL-101 (Fe) binary MOF/carboxylated-GO composite for adsorptive removal of methylene blue dye from aqueous solutions, RSC Adv., 2020, 10(32), 19008–19019 RSC.
- C. Shan, X. Zhang, S. Ma, X. Xia, Y. Shi and J. Yang, Preparation and application of bimetallic mixed ligand MOF photocatalytic materials, Colloids Surf., A, 2022, 636, 128108 CrossRef CAS.
- S. Ma, Y. Shi, X. Xia, Q. Song and J. Yang, Cerium-cobalt bimetallic metal–organic frameworks with the mixed ligands for photocatalytic degradation of methylene blue, Inorg. Chem. Commun., 2023, 152, 110664 CrossRef CAS.
- Q. Zhang, Y. Cheng, C. Liu and C. Fang, Electrochemical-driven removal of organic dyes by using bimetallic MOFs/waste cellulose acetate derived carbon foam as a freestanding electrode material, J. Solid State Chem., 2024, 330, 124489 CrossRef CAS.
- L. Feng, G. Ren, F. Wang, W. Yang, G. Zhu and Q. Pan, Two bimetallic metal–organic frameworks capable of direct photocatalytic degradation of dyes under visible light, Transit. Met. Chem., 2019, 44, 275–281 Search PubMed.
- Q. Sun, S. Huang, Z. Li, D. Su and J. Sun, Synergistic activation of persulfate by heat and cobalt-doped-bimetallic-MOFs for effective methylene blue degradation: synthesis, kinetics, DFT calculation, and mechanisms, J. Environ. Chem. Eng., 2023, 11(1), 109065 CrossRef CAS.
- G. Liu, Z. Liu, S. Li, C. Shi, T. Xu and M. Huo, et al., Aluminum copper bimetallic metal organic gels/sodium alginate beads for efficient adsorption of ciprofloxacin and methylene blue: adsorption isotherm, kinetic and mechanism studies, Process Saf. Environ. Prot., 2023, 176, 763–775 CrossRef CAS.
- H. Gupta, I. Saini, V. Singh, V. Singh, S. Yarramaneni and P. Grover, Fast decomposition of organic contaminant in wastewater using Zn and Mn bimetallic metal organic frameworks, Polyhedron, 2024, 117116 Search PubMed.
- F. Akbarbandari, M. Zabihi and M. Faghihi, Synthesis of the magnetic core–shell bi-metallic and tri-metallic metal–organic framework nanocomposites for dye adsorption, Water Environ. Res., 2021, 93(6), 906–920 CrossRef CAS PubMed.
- M. Zhong, S. Qu, K. Zhao, P. Fei, M. Wei and H. Yang, et al., Bimetallic Metal-Organic Framework Derived ZnO/Ni0.9Zn0.1O Nanocomposites for Improved Photocatalytic Degradation of Organic Dyes, ChemistrySelect, 2020, 5(6), 1858–1864 CrossRef CAS.
- Q. Chen, L. Tian, M. Ruan, Y. Liu, C. Peng and W. Cui, et al., HKUST-1/ZIF-67 nanocomposites as heterogeneous Cu–Co-bimetallic Fenton-like catalysts for efficient removal of methylene blue, ACS Appl. Nano Mater., 2024, 7(2), 2370–2381 Search PubMed.
- H. Li, Z. Yang, S. Lu, L. Su, C. Wang and J. Huang, et al., Nano-porous bimetallic CuCo-MOF-74 with coordinatively unsaturated metal sites for peroxymonosulfate activation to eliminate organic pollutants: performance and mechanism, Chemosphere, 2021, 273, 129643 CrossRef CAS PubMed.
- H. Li, C. Xu, N. Li, T. Rao, Z. Zhou and Q. Zhou, et al., Synthesis of bimetallic FeCu-MOF and its performance as catalyst of peroxymonosulfate for degradation of methylene blue, Materials, 2022, 15(20), 7252 CrossRef CAS PubMed.
- A. Siddiqa, T. Akhter, M. Faheem, S. Razzaque, A. Mahmood and W. Al-Masry, et al., Bismuth-rich Co/Ni bimetallic metal–organic frameworks as photocatalysts toward efficient removal of organic contaminants under environmental conditions, Micromachines, 2023, 14(5), 899 Search PubMed.
- S. Momin, T. Mahmood, A. Ullah, A. Naeem and A. Khan, Facile Synthesis of Cu–Zn Bimetallic MOF: Application as Superior Adsorbent for Effective Removal of Methylene Blue from Aqueous Solutions, Arabian J. Sci. Eng., 2023, 1–22 Search PubMed.
- J. Zheng, L. Sun, C. Jiao, Q. Shao, J. Lin and D. Pan, et al., Hydrothermally synthesized Ti/Zr bimetallic MOFs derived N self-doped TiO2/ZrO2 composite catalysts with enhanced photocatalytic degradation of methylene blue, Colloids Surf., A, 2021, 623, 126629 Search PubMed.
- J. Li, C. Yan, D. Sun, H. Ma, G. Wang and C. Ma, et al., Peroxymonosulfate activation by magnetic CoNi-MOF catalyst for degradation of organic dye, Environ. Sci. Pollut. Res., 2023, 30(27), 70371–70385 CrossRef CAS PubMed.
- L. Chen, X. Ren, N. S. Alharbi and C. Chen, Fabrication of a novel Co/Ni-MOFs@ BiOI composite with boosting photocatalytic degradation of methylene blue under visible light, J. Environ. Chem. Eng., 2021, 9(5), 106194 CrossRef CAS.
- P. Vatani, M. Aliannezhadi and F. S. Tehrani, Improvement of optical and structural properties of ZIF-8 by producing multifunctional Zn/Co bimetallic ZIFs for wastewater treatment from copper ions and dye, Sci. Rep., 2024, 14(1), 15434 CrossRef CAS PubMed.
- H. Wang, S. Yu, X. Meng, Z. Wang, T. Gao and S. Xiao, Facile synthesis of fumarate-type iron-cobalt bimetallic MOFs and its application in photo-Fenton degradation of organic dyes, J. Solid State Chem., 2022, 314, 123431 Search PubMed.
- T. Noor, U. Raffi, N. Iqbal, L. Yaqoob and N. Zaman, Kinetic evaluation and comparative study of cationic and anionic dyes adsorption on zeolitic imidazolate frameworks based metal organic frameworks, Mater. Res. Express, 2019, 6(12), 125088 CrossRef CAS.
- S. Kundu, B. Satpati, T. Kar and S. K. Pradhan, Microstructure characterization of hydrothermally synthesized PANI/V2O5·nH2O heterojunction photocatalyst for visible light induced photodegradation of organic pollutants and non-absorbing colorless molecules, J. Hazard. Mater., 2017, 339, 161–173 CrossRef CAS PubMed.
- M. Tuzen, A. Sarı and T. A. Saleh, Response surface optimization, kinetic and thermodynamic studies for effective removal of rhodamine B by magnetic AC/CeO2 nanocomposite, J. Environ. Manage., 2018, 206, 170–177 CrossRef CAS PubMed.
- B. Hu, J.-Y. Yuan, J.-Y. Tian, M. Wang, X. Wang and L. He, et al., Co/Fe-bimetallic organic framework-derived carbon-incorporated cobalt–ferric mixed metal phosphide as a highly efficient photocatalyst under visible light, J. Colloid Interface Sci., 2018, 531, 148–159 CrossRef CAS PubMed.
- Z. Xiao, R. Wu, T. Shu, Y. Wang and L. Li, Synthesis of Co-doped Fe metal–organic framework MIL-101 (Fe, Co) and efficient degradation of organic dyes in water, Sep. Purif. Technol., 2023, 304, 122300 CrossRef CAS.
- W. Cheng, Y. Wang, S. Ge, X. Ding, Z. Cui and Q. Shao, One-step microwave hydrothermal preparation of Cd/Zr-bimetallic metal–organic frameworks for enhanced photochemical properties, Adv. Compos. Hybrid Mater., 2021, 4, 150–161 CrossRef CAS.
- A. Mukherjee, P. Dhak and D. Dhak, The solvothermal synthesis of a 3D rod-like Fe–Al bimetallic metal–organic-framework for efficient fluoride adsorption and photodegradation of water-soluble carcinogenic dyes, Environ. Sci.: Adv., 2022, 1(2), 121–137 CAS.
- W. Liu, W. Duan, Q. Zhang, X. Gong and J. Tian, Novel bimetallic MOF derived N-doped carbon supported Ru nanoparticles for efficient reduction of nitro aromatic compounds and rhodamine B, New J. Chem., 2022, 46(35), 17004–17015 Search PubMed.
- M. S. Athar, A. Khan, I. Ahmad and M. Muneer, La/Fe bimetallic MOF-derived p-LaFeO 3/n-CdS heterojunction: efficient photocatalytic degradation of organic contaminants and adsorption isotherms, New J. Chem., 2024, 48(12), 5346–5361 Search PubMed.
- W. Xiang, X. Zhang, R. Xiao and Y. Zhang, Three-dimensional porous bimetallic metal-organic framework/gelatin aerogels: a readily recyclable peroxymonosulfate activator for efficient and continuous organic dye removal, Chin. J. Chem. Eng., 2024, 71, 193–202 CrossRef CAS.
- S. Patial, S. Thakur, Q. V. Le, T. Ahamad, P. Singh and V.-H. Nguyen, et al., Facile synthesis of Co, Fe-bimetallic MIL-88A/microcrystalline cellulose composites for efficient adsorptive and photo-Fenton degradation of RhB dye, J. Taiwan Inst. Chem. Eng., 2023, 153, 105189 CrossRef CAS.
- Z. Sun, X. Wu, K. Qu, Z. Huang, S. Liu and M. Dong, et al., Bimetallic metal-organic frameworks anchored corncob-derived porous carbon photocatalysts for synergistic degradation of organic pollutants, Chemosphere, 2020, 259, 127389 CrossRef PubMed.
- A. Fdez-Sanromán, E. Rosales, M. Pazos and A. Sanromán, One-pot synthesis of bimetallic Fe–Cu metal–organic frameworks composite for the elimination of organic pollutants via peroxymonosulphate activation, Environ. Sci. Pollut. Res., 2023, 1–16 Search PubMed.
- W. Lou, L. Wang, Z. Cao, Y. Zhang, J. Yang and L. Cheng, BiOBr as template and Bi3+ source to support the growth of Bi-Zn bimetallic MOF and hybrid photocatalysts with highly visible-light photocatalytic performances, J. Mater. Sci., 2023, 58(6), 2506–2524 CrossRef.
- R. Palani, V. Anitha, C. Karuppiah, S. Rajalakshmi, Y.-J. J. Li and T.-F. Hung, et al., Imidazolatic-framework bimetal electrocatalysts with a mixed-valence surface anchored on an rGO matrix for oxygen reduction, water splitting, and dye degradation, ACS Omega, 2021, 6(24), 16029–16042 CrossRef PubMed.
- A. Fdez-Sanromán, B. Lomba-Fernández, M. Pazos, E. Rosales and A. Sanromán, Peroxymonosulfate activation by different synthesized CuFe-MOFs: application for dye, drugs, and pathogen removal, Catalysts, 2023, 13(5), 820 CrossRef.
- T. K. N. Tran, H. L. Ho, H. V. Nguyen, B. T. Tran, T. T. Nguyen and P. Q. T. Bui, et al., Photocatalytic degradation of rhodamine B in aqueous phase by bimetallic metal-organic framework M/Fe-MOF (M= Co, Cu, and Mg), Open Chem., 2022, 20(1), 52–60 CrossRef.
- H.-T. T. Nguyen, K.-N. T. Tran, L. V. Tan, V. A. Tran, V.-D. Doan and T. Lee, et al., Microwave-assisted solvothermal synthesis of bimetallic metal-organic framework for efficient photodegradation of organic dyes, Mater. Chem. Phys., 2021, 272, 125040 CrossRef CAS.
- X. Pang, L. Sellaoui, D. Franco, M. S. Netto, J. Georgin and G. L. Dotto, et al., Preparation and characterization of a novel mountain soursop seeds powder adsorbent and its application for the removal of crystal violet and methylene blue from aqueous solutions, Chem. Eng. J., 2020, 391, 123617 CrossRef CAS.
- F. M. Jais, S. Ibrahim, C. Y. Chee and Z. Ismail, Solvothermal growth of the bimetal organic framework (NiFe-MOF) on sugarcane bagasse hydrochar for the removal of dye and antibiotic, J. Environ. Chem. Eng., 2021, 9(6), 106367 CrossRef CAS.
- M. Esteva, A. M. Ruiz and A. M. Stoka, Trypanosoma cruzi: methoprene is a potent agent to sterilize blood infected with trypomastigotes, Exp. Parasitol., 2002, 100(4), 248–251 CrossRef CAS.
- P. Li, Y.-J. Su, Y. Wang, B. Liu and L.-M. Sun, Bioadsorption of methyl violet from aqueous solution onto Pu-erh tea powder, J. Hazard. Mater., 2010, 179(1–3), 43–48 CrossRef CAS PubMed.
- L. R. Bonetto, F. Ferrarini, C. De Marco, J. S. Crespo, R. Guégan and M. Giovanela, Removal of methyl violet 2B dye from aqueous solution using a magnetic composite as an adsorbent, J. Water Process Eng., 2015, 6, 11–20 CrossRef.
- R. Xu, S. Xiao, J. Yuan and A. Zhao, Adsorption of methyl violet from aqueous solutions by the biochars derived from crop residues, Bioresour. Technol., 2011, 102(22), 10293–10298 CrossRef CAS.
- T. T. A. Le, B. H. Dang, T. Q. C. Nguyen, D. P. Nguyen and G. H. Dang, Highly efficient removal of tetracycline and methyl violet 2B from aqueous solution using the bimetallic FeZn-ZIFs catalyst, Green Process. Synth., 2023, 12(1), 20230122 CrossRef CAS.
- X. Shi, Y. Zu, S. Jiang and F. Sun, An anionic indium–organic framework with spirobifluorene-based ligand for selective adsorption of organic dyes, Inorg. Chem., 2021, 60(3), 1571–1578 CrossRef CAS.
- B. Zhang, Y. Wu and L. Cha, Removal of methyl orange dye using activated biochar derived from pomelo peel wastes: performance, isotherm, and kinetic studies, J. Dispersion Sci. Technol., 2020, 125–136 CrossRef.
- S. Bao, K. Li, P. Ning, J. Peng, X. Jin and L. Tang, Synthesis of amino-functionalization magnetic multi-metal organic framework (Fe3O4/MIL-101 (Al0.9Fe0.1)/NH2) for efficient removal of methyl orange from aqueous solution, J. Taiwan Inst. Chem. Eng., 2018, 87, 64–72 CrossRef CAS.
- T. Tang, X. Jin, X. Tao, L. Huang and S. Shang, Low-crystalline Ce-based bimetallic MOFs synthesized via DBD plasma for excellent visible photocatalytic performance, J. Alloys Compd., 2022, 895, 162452 CrossRef CAS.
- M. A. Nazir, M. F. Elsadek, S. Ullah, I. Hossain, T. Najam and S. Ullah, et al., Synthesis of bimetallic Mn@ ZIF–8 nanostructure for the adsorption removal of methyl orange dye from water, Inorg. Chem. Commun., 2024, 165, 112294 CrossRef CAS.
- M. A. Nazir, T. Najam, K. Zarin, K. Shahzad, M. S. Javed and M. Jamshaid, et al., Enhanced adsorption removal of methyl orange from water by porous bimetallic Ni/Co MOF composite: a systematic study of adsorption kinetics, Int. J. Environ. Anal. Chem., 2023, 103(16), 4841–4856 CrossRef CAS.
- F. M. Valadi, A. Ekramipooya and M. R. Gholami, Selective separation of Congo red from a mixture of anionic and cationic dyes using magnetic-MOF: experimental and DFT study, J. Mol. Liq., 2020, 318, 114051 CrossRef CAS.
- E. M. A. El-Monaem, A. M. Omer and A. S. Eltaweil, Durable and low-cost chitosan decorated Fe/MOF-5 bimetallic MOF composite film for high performance of the Congo red adsorption, J. Polym. Environ., 2024, 32(5), 2075–2090 CrossRef.
- Y. Liu, G. Qiu, Y. Liu, Y. Niu, R. Qu and C. Ji, et al., Fabrication of CoFe-MOF materials by different methods and adsorption properties for Congo red, J. Mol. Liq., 2022, 360, 119405 CrossRef CAS.
- X. Li, Z. Wu, X. Tao, R. Li, D. Tian and X. Liu, Gentle one-step co-precipitation to synthesize bimetallic CoCu-MOF immobilized laccase for boosting enzyme stability and Congo red removal, J. Hazard. Mater., 2022, 438, 129525 CrossRef CAS.
- S. Goyal, P. Ragui, A. Yadav, S. Rani, P. Dwivedi and R. K. Sharma, A facile synthesis of bimetallic Ni/Co-BTC hollow MOFs for effective removal of Congo red, Sep. Sci. Technol., 2024, 1–14 Search PubMed.
- B. Li, X. Zhang, J. Shen, A. Zhang and H. Huang, Bimetallic PCN-333 with Modulated Crystallization and a Porosity Structure for a Highly Efficient Removal of Congo Red, ACS Omega, 2024, 9(6), 7173–7187 CrossRef CAS.
- Q. Luo, X. Huang, Q. Deng, X. Zhao, H. Liao and H. Deng, et al., Novel 3D cross-shaped Zn/Co bimetallic zeolite imidazolate frameworks for simultaneous removal Cr (VI) and Congo red, Environ. Sci. Pollut. Res., 2022, 29(26), 40041–40052 CrossRef CAS PubMed.
- M. Yang and Q. Bai, Flower-like
hierarchical Ni-Zn MOF microspheres: efficient adsorbents for dye removal, Colloids Surf., A, 2019, 582, 123795 CrossRef CAS.
- H. Long, X. Li, X. Liu, W. Wang, X. Yang and Z. Wu, Immobilization of Laccase by Alkali-Etched Bimetallic CoCu-MOF To Enhance Enzyme Loading and Congo Red Degradation, Langmuir, 2023, 39(24), 8404–8413 CrossRef.
- N. Li, H. Li, R. Ji, S. Lin, C. Xu and J. Huang, et al., Fabrication of bimetallic MOF with 2D nanosheets structure and rich active sites for enhanced removal of organic pollutants by activation of peroxymonosulfate, J. Environ. Chem. Eng., 2023, 11(5), 110607 CrossRef.
- Z. Wang, J. Qi, B. Han, H. Jiang, M. He and J. Ma, Heterostructure engineered membranes based on two-dimensional bimetallic MOF for enhanced remediation of dye contaminated wastewater, J. Membr. Sci., 2024, 700, 122720 CrossRef.
- M. B. Nguyen, G. H. Le, T. D. Nguyen, Q. K. Nguyen, T. T. T. Pham and T. Lee, et al., Bimetallic Ag-Zn-BTC/GO composite as highly efficient photocatalyst in the photocatalytic degradation of reactive yellow 145 dye in water, J. Hazard. Mater., 2021, 420, 126560 CrossRef.
- H. M. Abumelha, Efficient removal of Sunset yellow food dye from aqueous environment using bimetal-organic frameworks encapsulated with chitosan: synthesis, characterization, adsorption analysis, and optimization, J. Mol. Liq., 2024, 125208 CrossRef.
- R. G. El-Sharkawy, A. S. B. El-Din and S. E. H. Etaiw, Kinetics and mechanism of the heterogeneous catalyzed oxidative decolorization of Acid-Blue 92 using bimetallic metal–organic frameworks, Spectrochim. Acta, Part A, 2011, 79(5), 1969–1975 CrossRef.
- S. Sallam, M. Aljohani, N. M. Alatawi, H. Alsharief, S. F. Ibarhiam and A. Almahri, et al., Box-Behnken design optimization of bimetallic-organic frameworks for effective removal of tartrazine food dye from aqueous solutions, J. Mol. Liq., 2024, 393, 123667 CrossRef CAS.
- V. Vatanpour, R. Ardic, B. Esenli, B. Eryildiz-Yesir, P. Y. Pazoki and A. Jarahiyan, et al., Defected Ag/Cu-MOF as a modifier of polyethersulfone membranes for enhancing permeability, antifouling properties and heavy metal and dye pollutant removal, Sep. Purif. Technol., 2024, 345, 127336 CrossRef CAS.
- X. Yang, J. Zhao, A. Cavaco-Paulo, J. Su and H. Wang, Encapsulated laccase in bimetallic Cu/Zn ZIFs as stable and reusable biocatalyst for decolorization of dye wastewater, Int. J. Biol. Macromol., 2023, 233, 123410 CrossRef CAS PubMed.
- S. Li, J. Tan, Z. Jiang, J. Wang and Z. Li, MOF-derived bimetallic Fe-Ni-P nanotubes with tunable compositions for dye-sensitized photocatalytic H2 and O2 production, Chem. Eng. J., 2020, 384, 123354 CrossRef CAS.
- S. Soni, N. Rai, P. K. Bajpai, J. Mittal and C. Arora, Enhanced sequestration of an acidic dye on novel bimetallic metal-organic framework, J. Dispersion Sci. Technol., 2023, 45(1), 107–116 CrossRef.
- F. S. Mustafa and A. A. Oladipo, Rapid degradation of anionic azo dye from water using visible light-enabled binary metal-organic framework, J. Water Process Eng., 2024, 64, 105686 CrossRef.
- M. Wang, L. Yang, C. Guo, X. Liu, L. He and Y. Song, et al., Bimetallic Fe/Ti-Based Metal–Organic Framework for Persulfate-Assisted Visible Light Photocatalytic Degradation of Orange II, ChemistrySelect, 2018, 3(13), 3664–3674 CrossRef CAS.
- J. Wang, C. Liu, J. Feng, D. Cheng, C. Zhang and Y. Yao, et al., MOFs derived Co/Cu bimetallic nanoparticles embedded in graphitized carbon nanocubes as efficient Fenton catalysts, J. Hazard. Mater., 2020, 394, 122567 CrossRef CAS.
- S. M. Mahmoud, S. H. Ammar, F. D. Ali and N. D. Ali, Fabrication and catalytic properties of polyoxometalates immobilized on mono-and bimetallic-zeolitic imidazolate frameworks for degradation of AV7 dye, J. Iran. Chem. Soc., 2023, 20(5), 1135–1144 CrossRef CAS.
- M. B. Nguyen, L. H. T. Nguyen, M. T. Le, N. Q. Tran, N. H. T. Tran and P. H. Tran, et al., Engineering direct Z-scheme GCN/bimetallic-MOF heterojunctions as efficient and recyclable photocatalysts for enhancing degradation of RR 195 under visible light, J. Ind. Eng. Chem., 2024, 134, 217–230 CrossRef CAS.
- J. Si, S. Zhang, X. Liu and K. Fang, Flower-shaped Ni/Co MOF with the highest adsorption capacity for reactive dyes, Langmuir, 2022, 38(19), 6004–6012 CrossRef CAS PubMed.
- M. Gharagozlou, N. E. Fard, M. Ghahari and M. T. Yaraki, Bimetal Cu/Ni-BTC@ SiO2 metal-organic framework as high performance photocatalyst for degradation of azo dyes under visible light irradiation, Environ. Res., 2024, 119229 CrossRef CAS.
- S. Kaushal, P. P. Singh and N. Kaur, Metal organic framework-derived Zr/Cu bimetallic photocatalyst for the degradation of tetracycline and organic dyes, Environ. Nanotechnol., Monit. Manage., 2022, 18, 100727 CAS.
- C. S. Budi, J. R. Deka, W.-C. Hsu, D. Saikia, K.-T. Chen and H.-M. Kao, et al., Bimetallic Co/Zn zeolitic imidazolate framework ZIF-67 supported Cu nanoparticles: an excellent catalyst for reduction of synthetic dyes and nitroarenes, J. Hazard. Mater., 2021, 407, 124392 CrossRef CAS.
- H. Xue, X.-S. Huang, Q. Yin, X.-J. Hu, H.-Q. Zheng and G. Huang, et al., Bimetallic cationic metal–organic frameworks for selective dye adsorption and effective Cr2O72–removal, Cryst. Growth Des., 2020, 20(8), 4861–4866 CrossRef.
- Q. Wu, M. S. Siddique and W. Yu, Iron-nickel bimetallic metal-organic frameworks as bifunctional Fenton-like catalysts for enhanced adsorption and degradation of organic contaminants under visible light: kinetics and mechanistic studies, J. Hazard. Mater., 2021, 401, 123261 CrossRef PubMed.
- L. Jin, X. Zhao, X. Qian and M. Dong, Nickel nanoparticles encapsulated in porous carbon and carbon nanotube hybrids from bimetallic metal-organic-frameworks for highly efficient adsorption of dyes, J. Colloid Interface Sci., 2018, 509, 245–253 CrossRef PubMed.
- F. Khosravi, M. Gholinejad, J. M. Sansano and R. Luque, Bimetallic Fe–Cu metal organic frameworks for room temperature catalysis, Appl. Organomet. Chem., 2022, 36(7), e6749 CrossRef.
- P. Lei, Y. Zhou, C. Dong, Y. Liu and S. Shuang, CoNi–MOF–graphene magnetic nanocomposites for the electrocatalytic detection of glucose and the efficient removal of organic dyes, ACS Appl. Nano Mater., 2023, 6(11), 9369–9375 CrossRef.
- T. K. N. Tran, C. P. K. Phan, T. C. Q. Ngo, N. B. Hoang, L. D. Truong and T. K. O. Nguyen, Synthesis and characterization bimetallic organic framework CoxFex (BDC) and adsorption cationic and anionic dyes, Processes, 2022, 10(7), 1352 CrossRef.
- H. S. Far, M. Hasanzadeh, M. Najafi and M. Rabbani, Highly porous organoclay-supported bimetal–organic framework (CoNi-MOF/OC) composite with efficient and selective adsorption of organic dyes, Environ. Sci. Pollut. Res., 2023, 30(15), 43714–43725 CrossRef CAS PubMed.
- X. Yang, X. Chen, H. Wang, A. Cavaco-Paulo and J. Su, Co-immobilizing laccase-mediator system by in situ synthesis of MOF in PVA hydrogels for enhanced laccase stability and dye decolorization efficiency, J. Environ. Manage., 2024, 353, 120114 CrossRef CAS PubMed.
|
This journal is © The Royal Society of Chemistry 2024 |
Click here to see how this site uses Cookies. View our privacy policy here.