DOI:
10.1039/D4RA07102F
(Paper)
RSC Adv., 2024,
14, 39543-39549
Electrophilic aromatic substitution using fluorinated isoxazolines at the C5 position via C–F bond cleavage†
Received
3rd October 2024
, Accepted 11th December 2024
First published on 16th December 2024
Abstract
Electrophilic aromatic substitution at the C5 position of isoxazolines and construction of a new quaternary carbon center were achieved in this paper. This is the first report of carbon–carbon (C–C) bond formation onto isoxazoline without compromising the ring structure. Various aromatics including heteroaromatics gave the desired products in good yields, especially aromatics bearing electron-donating groups. The reaction proceeds via the SEAr reaction mechanism, in which carbocation intermediates generated from the fluorinated isoxazolines via C–F bond cleavage reacted with aromatics.
Introduction
Heterocycles are important frameworks that are widely distributed in nature. The isoxazoline pharmacophore is one of the most important classes of five-membered nitrogen–oxygen containing heterocyclic compounds.1 Acivicin is a fermentation product of Streptomyces sviceus and used as an effective inhibitor of γ-glutamyl transferase (Fig. 1).2 Isoxadifen-ethyl is used as a herbicide safener that minimizes the effect of the herbicide, and can effectively alleviate a sulfonylurea herbicide ‘nicosulfuron’ injury in maize.3 Roxifiban is a selective antagonist of the platelet glycoprotein IIb/IIIa receptor, which is the major receptor for fibrinogen on the platelet surface.4 Among such isoxazoline scaffolds, in particular, fluorinated or fluoroalkylated derivatives exhibit remarkable biological properties. For example, fluxametamide is an insecticide with a wide spectrum, and acts as an antagonist of GABA- and glutamate-gated chloride channels (GABA-Cl and Glu-Cl).5 Furthermore, CBM-301940 exhibited excellent in vivo PK/ADME properties and improved the cardiac efficiency in a rat heart global ischemia/reperfusion model.6
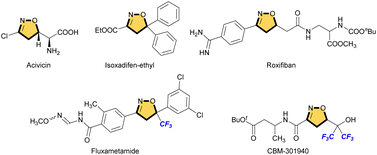 |
| Fig. 1 Isoxazolines in bio-active compounds. | |
These scaffolds are commonly synthesized from fluorinated and/or fluoroalkylated building blocks which include classical reactions such as cycloaddition of nitrile oxide with alkene, condensation of diketone with hydroxylamine, and cyclization of oxime (Fig. 2).7 However, both 4-fluorinated and 4-fluoroalkylated isoxazolines scaffolds have been little synthesized, especially isoxazolines bearing a quaternary carbon center at C5 position, which is important for the expression of biological activity. The Khisamutdinov group and the Shibata group succeeded in fluorination of isoxazolines bearing electron-withdrawing group at C4, respectively.8 These reactions afforded the corresponding 4-fluorinated products in good yields, but the formation of a new quaternary carbon center was not achieved owing to the use of starting substances that already have substituents at the C5 position (Scheme 1a and b). In 2020, an interesting fluoro-spirocyclization of isoxazoles was reported by Hamme and his co-workers.9 They also succeeded in the synthesis of 4-fluorinated isoxazolines, and achieved the construction of a new quaternary carbon center at C5 via C–O bond formation as well (Scheme 1c). To the best of our knowledge, there are only three reports regarding fluorine-containing isoxazolines at C4 position, although Houk et al. also reported the [3 + 2] cycloaddition of nitrile oxides to give the related isoxazoline system, but those were not main products.10
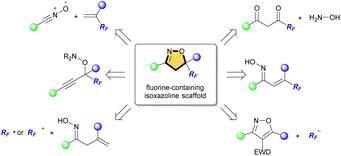 |
| Fig. 2 Synthetic methods for fluorine-containing isoxazolines. | |
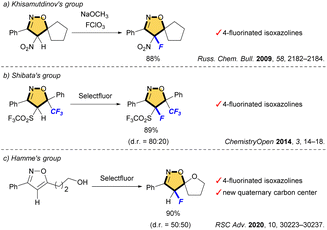 |
| Scheme 1 Synthesis of 4-fluorinated isoxazolines bearing a quaternary carbon at C5. | |
On the other hand, a carbon–fluorine (C–F) bond is one of the most stable chemical bonds, because it has the highest bond dissociation energies.11 For this reason, transformations through stable C–F bond cleavage are difficult, but also challenging reactions. In recent years, several C–F bond cleavage reactions have been achieved by some groups, but there is still room in this field.12 Based on such important background, we are interested in the construction of fluorinated isoxazoline system bearing a quaternary carbon center at C5 by using stable C–F bonds cleavage aimed at synthesizing a wide range of functional fluorinated 5-membered heterocyclic systems.
We reported selective fluorination of isoxazoles (1), which gave 4-fluorinated isoxazoles (2) or 4,4,5-trifluorinated isoxazolines (3) by using different amounts of Selectfluor, respectively (Scheme 2).13 Furthermore, when isoxazolines 3 and various alcohols were treated with SnCl4, the corresponding 5-alkoxylated products 4 were obtained in moderate to good yields (Scheme 3a).14 This reaction proceeds via an SN1 type process along with C–F bond cleavage, and then C–O bond formation by alcohol formed new quaternary carbon center at C5 position. In addition, this reaction could apply to other hetero atom nucleophiles such as thiols or amines to generate a new C–S or C–N bond at C5 position on starting isoxazolines.15 During the process of the synthesis of 4, sterically demanding phenol such as 2,6-diphenylphenol gave a novel aryl substituted product 5aA via electrophilic aromatic substitution (SEAr) as shown in Scheme 3b.14 Furthermore, the reaction with N,N-dimethylaniline gave the similar C–C bond forming product 5aD that was introduced the aromatic ring directly at the C5 position of the isoxazoline scaffold, although the yield should be improved as shown in Scheme 3c. This is the first report for introducing carbon nucleophiles directly at the C5 position of the isoxazoline ring without compromising ring structure. In view of the results, we made the following hypothesis that the reaction of 3 with aromatic compounds might give various 4,4-difluoro-5-arylated isoxazolines (5) via SEAr type processes which was directly constructed C–C bond at C5 position of isoxazolines (Scheme 4).
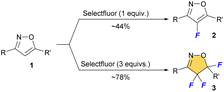 |
| Scheme 2 One-pot synthesis and selective fluorination of isoxazoles. | |
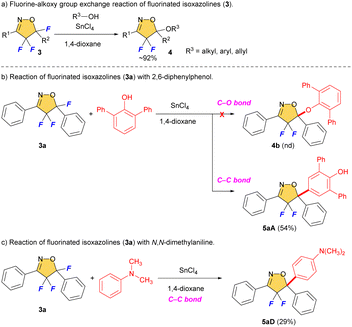 |
| Scheme 3 Fluorine-alkoxy group exchange reaction of fluorinated isoxazolines and unexpected SEAr products. | |
 |
| Scheme 4 Direct introduction of carbon nucleophiles on fluorinated isoxazolines via C–F bond cleavage. | |
Results and discussion
For introducing an aromatic ring at the C5 position of isoxazoline 3a, we investigated the reaction conditions by using toluene as a carbon nucleophile. According to the previous result, we applied the best condition of the fluorine-alkoxy group exchange reaction as shown in entry 1 (Table 1).14 However, compound 5aB was not obtained, but the main product is 5-hydroxylated compound 4a in 79%. Therefore, various reaction solvents were examined. As shown in entries 4 and 6, acetonitrile and sulfolane gave the desired product 5aB, and we found that sulfolane is the best solvent in this reaction. Next, various Lewis acids were examined. As shown in entry 7, using LiCl did not give the product at all, and only the starting material 3a was recovered. On the other hand, using other Lewis acids such as YbCl3, FeCl3, SnCl2 or TiCl4 gave 5-hydroxylated product 4a, although the 3a was consumed as shown in entries 9–12. Based on these examinations, BF3·Et2O was the best Lewis acid and it gave the desired product 5aB in 58% (entry 8). In further optimization of the reaction conditions, the solution concentrations and the temperature were investigated. In entry 13, increasing the solution concentration improved the yield of 5aB. However, further high concentration or high reaction temperature greatly decreased the yield as shown in entries 14 and 15. On the other hand, the shortened reaction time did not affect the reaction yield (entry 16). So, we decided entry 16 is the best condition to give the desired product 5aB.
Table 1 Reaction conditions for SEAr using toluene

|
Entry |
Lewis acid |
Solv. (mol L−1) |
Temp (°C) |
Yielda (%) |
3a |
4a |
5aB |
Isolated yield. The yield in parentheses was calculated by 19F NMR using PhCF3 as an internal standard. The reaction was carried out for 1 h. |
1 |
SnCl4 |
14-Dioxane (0.125) |
Reflux |
Trace |
79 |
Trace |
2 |
SnCl4 |
DCE (0.125) |
Reflux |
(34)b |
47 |
— |
3 |
SnCl4 |
THE (0.125) |
Reflux |
(23)b |
(20)b |
— |
4 |
SnCl4 |
CH3CN (0.125) |
Reflux |
— |
57 |
18 |
5 |
SnCl4 |
DMF (0.125) |
90 |
82 |
Trace |
— |
6 |
SnCl4 |
Sulfolane (0.125) |
90 |
— |
Trace |
37 |
7 |
LiCl |
Sulfolane (0.125) |
90 |
96 |
— |
— |
8 |
BF3·Et2O |
Sulfolane (0.125) |
90 |
— |
— |
58 |
9 |
TiCl4 |
Sulfolane (0.125) |
90 |
— |
19 |
— |
10 |
FeC13·6H2O |
Sulfolane (0.125) |
90 |
— |
61 |
— |
11 |
SnCl2 |
Sulfolane (0.125) |
90 |
— |
98 |
— |
12 |
YbC13·6H2O |
Sulfolane (0.125) |
90 |
— |
94 |
— |
13 |
BF3·Et2O |
Sulfolane (0.125) |
90 |
— |
— |
66 |
14 |
BF3·Et2O |
Sulfolane (0.5) |
90 |
— |
— |
27 |
15 |
BF3·Et2O |
Sulfolane (0.125) |
120 |
— |
— |
12 |
16c |
BF3·Et2O |
Sulfolane (0.125) |
90 |
— |
— |
64 |
On the basis of the optimized conditions, we explored the scope for this reaction, and the results are summarized in Table 2. The aromatics that are bearing on electron-donating groups proceeded smoothly to give the corresponding compounds (5aB–5aD, 5aH and 5aI) in moderate to good yields. On the other hand, the electron-deficient aromatics such as bromobenzene, benzotrifluoride and acetophenone did not work well, but only 5-hydroxylated product 4a was identified on 19F NMR. Heteroaromatic compounds could be applied to this reaction. Using N-methylpyrrole as the substrate, the desired compound 5aJ was obtained in 43% together with its regioisomer 5aJ′ in 43% yield. Fortunately, these isomers (5aJ and 5aJ′) were separable by column chromatography and the total yield of this reaction was 86%. With furan and thiophene, the reaction gave rise to the corresponding SEAr products (5aK and 5aL) including a small amount of their regioisomers in 54% and 86% yields. However, pyridine did not give the product at all, but the only starting material 3a was recovered. Pyridine is a basic amine, so the formation of a BF3 salt might be predominated during the reaction. Isoxazolines having substituted aromatics and alkyl group also reacted with toluene to give the corresponding products (5bB, 5cB, and 5dB). It is interesting that 5cB involving chlorophenyl group at C5 could be obtained in a good yield by using the corresponding starting material, although the introduction of halobenzene via the SEAr reaction failed (see 5aE). As a side note, the molecular structures of 5aH, 5aI and 5aJ were characterized by using single-crystal XRD analyses (5aH (CCDC: 2349494), 5aI (CCDC: 2349492) and 5aJ (CCDC: 2349493)).
Table 2 SEAr reaction of 4,4,5-trifluorinated isoxazolines with aromatics via C–F bond cleavage
The product and the starting material (3a) were identified on 19F NMR. Only the 5-hydroxylated product (4aA) was identified on 19F NMR. Separable regioisomer (5aJ′) was obtained in 43%. lnseparable regioisomer was obtained in a small amount. The starting material (3a) was recovered. |
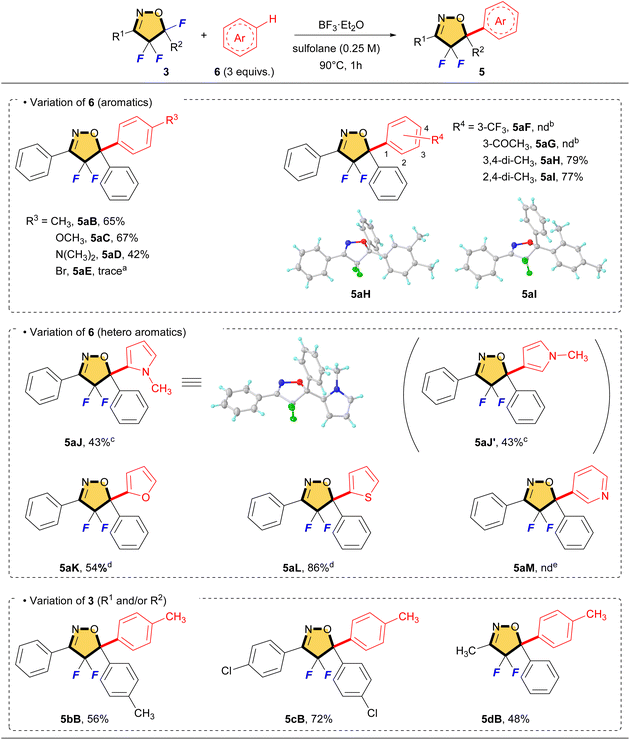 |
We envisage that the reaction proceeds via SEAr reaction mechanism (Fig. 3). The C–F bond of 3a would be dissociated by Lewis acid and give the carbocation intermediate A (Int A). The stabilized Int A by the adjacent oxygen atom and/or benzene ring was trapped by the aromatics via SEAr mechanism to give 5-arylated product 5 that bears a new quaternary carbon center. On the other hand, using poorly reactive electron-deficient aromatics preferentially led to the trapping of sulfolane and/or H2O towards Int A to give 5-hydroxylated product 4a reflecting the lower nucleophilicity of the aromatic substrate bearing an electron withdrawing substituent. So, it is important to use ‘dry’ solvent in this reaction.
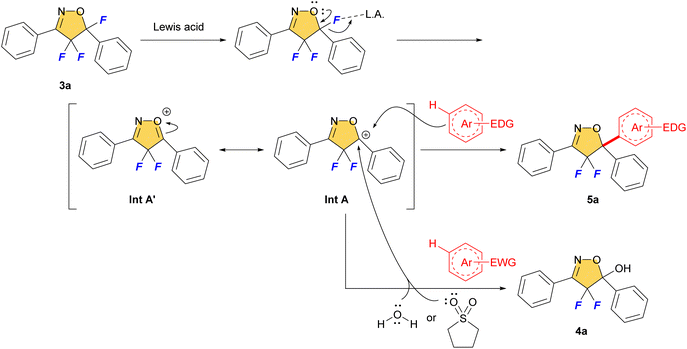 |
| Fig. 3 Proposed reaction mechanism of SEAr reaction. | |
In the last part, we explored several constructive reactions to demonstrate the synthetic utility of fluorinated isoxazoline products (Scheme 5). A reductive N–O bond cleavage of 5aB followed by hydrolysis of imine to give α,α-difluoro-β-hydroxy ketone 6 in 68% yield.16 Furthermore, treatment of 5bB under reductive ring-opening condition by NaBH4 and NiCl2 afforded the corresponding α,α-difluoro-β-amino alcohol 7 in 62% yield.17 Interestingly, these are the first synthetic examples of α,α-difluoro-β-hydroxy ketone and α,α-difluoro-β-amino alcohol bearing two aromatic rings on the quaternary carbon center, although many syntheses of similar compounds using Reformatsky-type reaction and/or aldol-type reaction have been reported.18
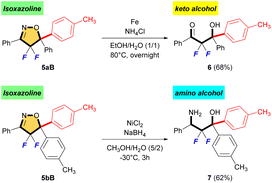 |
| Scheme 5 The synthetic utility of fluorinated isoxazolines. | |
Conclusions
In conclusion, we succeeded in introducing various aromatic substituents at the C5 position of the fluorinated isoxazolines. Using the aromatics bearing electron-donating groups and heteroaromatics gave the desired products 5 in good yields. On the other hand, electron deficient aromatics did not give the products. The reaction would proceed via the SEAr reaction mechanism, in which carbocation intermediates generated from the fluorinated isoxazolines via stable C–F bond cleavage reacted with aromatics. Isoxazoline is an important framework for bioactive compounds, and we expect that these products also have interesting activities.
Data availability
All experimental procedures and additional data can be found in the ESI.†
Author contributions
KS wrote the manuscript. KS and MO conceived and designed the experiments. KS, TK and HM performed the experiments and analyzed the data. AS carried out the single-crystal XRD analyses. All authors discussed the results and reviewed the manuscript.
Conflicts of interest
There are no conflicts to declare.
Acknowledgements
We thank Dr Haruhiko Fukaya at Tokyo University of Pharmacy and Life Sciences for support with X-ray crystallographic studies.
Notes and references
-
(a) K. A. Kumar, M. Govindaraju, N. Renuka and G. V. Kumar, Isoxazolines: An insight to their synthesis and diverse applications, Int. J. Res. Pharm. Chem., 2015, 7, 250–257 Search PubMed;
(b) N. Agrawal and P. Mishra, The synthetic and therapeutic expedition of isoxazole and its analogs, Med. Chem. Res., 2018, 27, 1309–1344 CrossRef;
(c) G. Kumar and R. Shankar, 2-Isoxazolines: A Synthetic and Medicinal Overview, ChemMedChem, 2021, 16, 430–447 CrossRef;
(d) I. L. Gonçalves, G. Machado das Neves, L. P. Kagami, V. L. Eifler-Lima and A. A. Merlo, Discovery, development, chemical diversity and design of isoxazoline-based insecticides, Bioorg. Med. Chem., 2021, 30, 115934 CrossRef PubMed;
(e) D. Feng, S. Wu, B. Jiang, S. He, Y. Luo, F. Li, B. Song and R. Song, Discovery of novel isoxazoline derivatives containing diaryl ether against fall armyworms, J. Agric. Food Chem., 2023, 71, 6859–6870 CrossRef;
(f) S. Huang, H. Ma, Z. Wang, P. Zhang, S. Li, Y. Li, A. Liu, Y. Li and Q. Wang, Design, synthesis, and insecticidal and fungicidal activities of ether/oxime-ether containing isoxazoline derivatives, J. Agric. Food Chem., 2023, 71, 5107–5116 CrossRef PubMed.
- L. Allen, R. Meck and A. Yunis, The inhibition of gamma-glutamyl transpeptidase from human pancreatic carcinoma cells by (alpha S,5S)-alpha-amino-3-chloro-4,5-dihydro-5-isoxazoleacetic acid (AT-125; NSC-163501), Res. Commun. Chem. Pathol. Pharmacol., 1980, 27, 175–182 Search PubMed.
-
(a) H. Ahrens, G. Lange, T. Müller, C. Rosinger, L. Willms and A. van Almsick, 4-Hydroxyphenylpyruvate dioxygenase inhibitors in combination with safeners: Solutions for modern and sustainable agriculture, Angew Chem. Int. Ed. Engl., 2013, 52, 9388–9398 CrossRef PubMed;
(b) L. Sun, R. Wu, W. Su, Z. Gao and C. Lu, Physiological basis for isoxadifen-ethyl induction of nicosulfuron detoxification in maize hybrids, PLoS One, 2017, 12, e0173502 CrossRef PubMed.
-
(a) C.-B. Xue, J. Wityak, T. M. Sielecki, D. J. Pinto, D. G. Batt, G. A. Cain, M. Sworin, A. L. Rockwell, J. J. Roderick, S. Wang, M. J. Orwat, W. E. Frietze, L. L. Bostrom, J. Liu, C. A. Higley, F. W. Rankin, A. E. Tobin, G. Emmett, G. K. Lalka, J. Y. Sze, S. V. DiMeo, S. A. Mousa, M. J. Thoolen, A. L. Racanelli, E. A. Hausner, T. M. Reilly, W. F. DeGrado, R. R. Wexler and R. E. Olson, Discovery of an orally active series of isoxazoline glycoprotein IIb/IIIa antagonists, J. Med. Chem., 1997, 40, 2064–2084 CrossRef PubMed;
(b) C.-B. Xue and S. A. Mousa, DMP 754, Drugs Future, 1998, 23, 707–711 CrossRef.
- M. Asahi, M. Kobayashi, T. Kagami, K. Nakahira, Y. Furukawa and Y. Ozoe, Fluxametamide: A novel isoxazoline insecticide that acts via distinctive antagonism of insect ligand-gated chloride channels, Pestic. Biochem. Physiol., 2018, 151, 67–72 CrossRef PubMed.
- J.-F. Cheng, Y. Huang, R. Penuliar, M. Nishimoto, L. Liu, T. Arrhenius, G. Yang, E. O'Leary, M. Barbosa, R. Barr, J. R. B. Dyck, G. D. Lopaschuk and A. M. Nadzan, Discovery of potent and orally available malonyl-CoA decarboxylase inhibitors as cardioprotective agents, J. Med. Chem., 2006, 49, 4055–4058 CrossRef.
-
(a) K. Matoba, H. Kawai, T. Furukawa, A. Kusuda, E. Tokunaga, S. Nakamura, M. Shir and N. Shibata, Enantioselective synthesis of trifluoromethyl-substituted 2-isoxazolines: Asymmetric hydroxylamine/enone cascade reaction, Angew. Chem., Int. Ed., 2010, 49, 5762–5766 CrossRef;
(b) H. Kawai, K. Tachi, E. Tokunaga, M. Shiro and N. Shibata, Trifluoromethylation of aromatic isoxazoles: Regio- and diastereoselective route to 5-trifluoromethyl-2-isoxazolines, Angew. Chem., Int. Ed., 2011, 50, 7803–7806 CrossRef;
(c) V. Kumar and K. Kaur, Fluorinated isoxazolines and isoxazoles: A synthetic perspective, J. Fluorine Chem., 2015, 180, 55–97 CrossRef;
(d) J. Zhao, M. Jiang and J.-T. Liu, Synthesis of fluoromethyl-substituted isoxazolines via transition metal-free oxyfluorination of alkenyl oximes, Adv. Synth. Catal., 2017, 359, 1626–1630 CrossRef;
(e) N. Umemoto, A. Imayoshi and K. Tsubaki, Nitrile oxide cycloaddition reactions of alkenes or alkynes and nitroalkanes substituted with O-alkyloxime groups convertible to various functional groups, Tetrahedron Lett., 2020, 61, 152213 CrossRef;
(f) A. Sperga, A. Kazia and J. Veliks, Monofluorinated 5-membered rings via fluoromethylene transfer: synthesis of monofluorinated isoxazoline N-oxides, Org. Biomol. Chem., 2021, 19, 2688–2691 RSC;
(g) W. Riley, A. C. Jones, K. Singh, D. L. Browne and A. M. Stuart, Accessing novel fluorinated heterocycles with the hypervalent fluoroiodane reagent by solution and mechanochemical synthesis, Chem. Commun., 2021, 57, 7406–7409 RSC;
(h) M. Yang, W. Shao, L. Zuo, J. Wang, Y. Xu, G. Mao and G.-J. Deng, Site-selective radical trifluoromethylaminoxylation of olefins for the modular synthesis of diverse β-trifluoromethyl trisubstituted hydroxylamines and beyond, Org. Lett., 2023, 25, 2728–2732 CrossRef.
-
(a) G. Kh. Khisamutdinov, V. Okhlobystina and A. A. Fainzil’berg, Fluorination of 4-nitroisoxazoline salts. Synthesis of 4-fluoro-4-nitroisoxazolines and 4-fluoroisoxazoles, Russ. Chem. Bull., 2009, 58, 2182–2184 CrossRef;
(b) H. Kawai, Y. Sugita, E. Tokunaga, H. Sato, M. Shiro and N. Shibata, Diastereoselective additive trifluoromethylation/halogenation of isoxazole triflones: Synthesis of all-carbon-functionalized trifluoromethyl isoxazoline triflones, Open Chem., 2014, 3, 14–18 Search PubMed.
- P. Das, S. Boone, D. Mitra, L. Turner, R. Tandon, D. Raucher and A. T. Hamme II, Synthesis and biological evaluation of fluoro-substituted spiro-isoxazolines as potential anti-viral and anti-cancer agents, RSC Adv., 2020, 10, 30223–30237 RSC.
-
(a) Y.-M. Chang, J. Sims and K. N. Houk, Mechanisms of 1.3-dipolar cycloadditions to highly electron-deficient dipolarophiles, Tetrahedron Lett., 1975, 16, 4445–4448 CrossRef;
(b) M. Prakesch, D. Grée, R. Grée, J. Carter, I. Washington and K. N. Houk, Stereoselectivity of nitrile oxide cycloadditions to chiral allylic fluorides: Experiment and theory, Chem.–Eur. J., 2003, 9, 5664–5672 CrossRef PubMed.
- Y. G. Lazarou, A. V. Prosmitis, V. C. Papadimitriou and P. Papagiannakopoulos, Theoretical calculation of bond dissociation energies and enthalpies of formation for halogenated molecules, J. Phys. Chem. A, 2001, 105, 6729–6742 CrossRef.
-
(a) T. Niwa, H. Ochiai, Y. Watanabe and T. Hosoya, Ni/Cu-Catalyzed defluoroborylation of fluoroarenes for diverse C–F bond functionalizations, J. Am. Chem. Soc., 2015, 137, 14313–14318 CrossRef PubMed;
(b) T. Iwasaki, K. Yamashita, H. Kuniyasu and N. Kambe, Co-Catalyzed cross-coupling reaction of alkyl fluorides with alkyl Grignard reagents, Org. Lett., 2017, 19, 3691–3694 CrossRef;
(c) N. Sugihara, K. Suzuki, Y. Nishimoto and M. Yasuda, Photoredox-catalyzed C–F bond allylation of perfluoroalkylarenes at the benzylic position, J. Am. Chem. Soc., 2021, 143, 9308–9313 CrossRef;
(d) F. Wang, Y. Nishimoto and M. Yasuda, Insertion of diazo esters into C–F bonds toward diastereoselective one-carbon elongation of benzylic fluorides: Unprecedented BF3 catalysis with C–F bond cleavage and re-formation, J. Am. Chem. Soc., 2021, 143, 20616–20621 CrossRef PubMed;
(e) J. Zhou, B. Jiang, Y. Fujihira, Z. Zhao, T. Imai and N. Shibata, Catalyst-free carbosilylation of alkenes using silyl boronates and organic fluorides via selective C-F bond activation, Nat. Commun., 2021, 12, 3749 CrossRef PubMed;
(f) T. Ma, X.-P. Wei, X.-C. Wang, X.-X. Qiao, G. Li, Y. He and X.-J. Zhao, Highly enantioselective synthesis of 3a-fluorofuro[3,2-b]indolines via organocatalytic aza-Friedel–Crafts reaction/selective C–F bond activation, Org. Lett., 2023, 25, 8666–8671 CrossRef.
- K. Sato, G. Sandford, K. Shimizu, S. Akiyama, M. J. Lancashire, D. S. Yufit, A. Tarui, M. Omote, I. Kumadaki, S. Harusawa and A. Ando, Synthesis of fluorinated isoxazoles using Selectfluor™: preparation and characterization of 4-fluoroisoxazole, 4,4,5-trifluoroisoxazoline and 4,4-difluoro-5-hydroxyisoxazoline systems from one-pot and multi-step processes, Tetrahedron, 2016, 72, 1690–1698 CrossRef.
- K. Sato, G. Sandford, Y. Konishi, N. Yanada, C. Toda, A. Tarui and M. Omote, Lewis acid promoted fluorine-alkoxy group exchange reactions for the synthesis of 5-alkoxy-4,4-difluoroisoxazoline systems, Org. Biomol. Chem., 2019, 17, 2818–2823 RSC.
- K. Sato, K. Nakano, T. Ueda, Y. Kitamura, Y. Tanaka, Y. Karuo, A. Tarui, K. Kawai and M. Omote, Construction of new quaternary carbon center of fluorinated isoxazolines at C5 position via C-F bond cleavage, J. Fluorine Chem., 2024, 279, 110335 CrossRef.
- L. Wang, K. Zhang, Y. Wang, W. Li, M. Chen and J. Zhang, Enantioselective synthesis of isoxazolines enabled by palladium-catalyzed carboetherification of alkenyl oximes, Angew. Chem., Int. Ed., 2020, 59, 4421–4427 CrossRef.
- X.-T. Li, Q.-S. Gu, X.-Y. Dong, X. Meng and X.-Y. Liu, A copper catalyst with a cinchona-alkaloid-based sulfonamide ligand for asymmetric radical oxytrifluoromethylation of alkenyl oximes, Angew. Chem., Int. Ed., 2018, 57, 7668–7672 CrossRef PubMed.
-
(a) S. Sasaki, T. Suzuki, T. Uchiya, S. Toyota, A. Hirano, M. Tanemura, H. Teramoto, T. Yamauchi and K. Higashiyama, Synthesis of α,α-difluoro-β-hydroxy ketone via the La(OTf)3-catalyzed aldol reaction of carbonyl compounds with difluoroenol O-Boc esters, J. Fluorine Chem., 2016, 192, 78–85 CrossRef;
(b) A. Tarui, M. Oduti, S. Shinya, K. Sato and M. Omote, Decarboxylative aldol reaction of α,α-difluoro-β-ketocarboxylate salt: a facile method for generation of difluoroenolate, RSC Adv., 2018, 8, 20568–20575 RSC;
(c) X.-S. Hu, P.-G. Ding, J.-S. Yu and J. Zhou, A Sc(OTf)3 catalyzed Mukaiyama–Mannich reaction of difluoroenoxysilanes with unactivated ketimines, Org. Chem. Front., 2019, 6, 2500–2505 RSC;
(d) Z. Ni, R. Sun, J. Wu, C. Xu, J. Huang and F. Wu, Indium-mediated 1,2-addition of iododifluoromethyl ketones with α,β-unsaturated ketones, Tetrahedron, 2022, 119, 132855 CrossRef;
(e) R. Sun, X. Lian, J. Huang, J. Wu, Y. Li, Z. Chen, Z. Qu, L. Song and F. Wu, Iron-mediated Reformatsky reaction of iododifluoromethyl ketones with aldehydes: Preparation of α,α-difluoro-β-hydroxyketone derivatives, J. Fluorine Chem., 2023, 269, 110151 CrossRef;
(f) F. Livernaux, S. Lavieville, P. Guiffrey, J.-M. Campagne and E. Leclerc, Enantioselective aldol reaction with difluoroenoxysilanes catalyzed by cationic palladium aqua complexes, Eur. J. Org Chem., 2023, 26, e202300467 CrossRef.
|
This journal is © The Royal Society of Chemistry 2024 |
Click here to see how this site uses Cookies. View our privacy policy here.