DOI:
10.1039/D3SC05879D
(Edge Article)
Chem. Sci., 2024,
15, 3290-3299
Multiple control of azoquinoline based molecular photoswitches†
Received
3rd November 2023
, Accepted 18th January 2024
First published on 19th January 2024
Abstract
Multi-addressable molecular switches with high sophistication are creating intensive interest, but are challenging to control. Herein, we incorporated ring–chain dynamic covalent sites into azoquinoline scaffolds for the construction of multi-responsive and multi-state switching systems. The manipulation of ring–chain equilibrium by acid/base and dynamic covalent reactions with primary/secondary amines allowed the regulation of E/Z photoisomerization. Moreover, the carboxyl and quinoline motifs provided recognition handles for the chelation of metal ions and turning off photoswitching, with otherwise inaccessible Z-isomer complexes obtained via the change of stimulation sequence. Particularly, the distinct metal binding behaviors of primary amine and secondary amine products offered a facile way for modulating E/Z switching and dynamic covalent reactivity. As a result, multiple control of azoarene photoswitches was accomplished, including light, pH, metal ions, and amine nucleophiles, with interplay between diverse stimuli further enabling addressable multi-state switching within reaction networks. The underlying structural and mechanistic insights were elucidated, paving the way for the creation of complex switching systems, molecular assemblies, and intelligent materials.
Introduction
As one of the most employed photochromic switches, azobenzene has received unabated attention due to the simple structure, facile synthesis, large geometric differences between E/Z isomers, and high fatigue-resistance.1–4 In particular, the change in the molecular shape of azobenzene upon photoactivation offers a powerful strategy for manipulating guest recognition,5–8 molecular machines,9–12 supramolecular assemblies,13–16 and nanoparticles.17–20 Azoheteroarenes obtained by replacing one or both phenyl with a heteroaryl,21–24 such as pyridine,25–28 pyrazole,29–31 imidazole,32–34 and thiazole,35–37 are recently generating strong interest. Many research efforts have focused on the extension of new heterocycles and the modulation of their photoswitching properties through substituent effects.38,39 However, six-membered ring heterocycles beyond pyridine have been less studied.40–42 Azoquinoline has a phenyl directly attached to the azo group, but with an adjacent fused pyridyl group, which makes it intermediate in nature between azobenzene and azoheteroarene. In this context, photoinduced E → Z isomerization and thermal Z → E isomerization of azo compounds derived from hydrogenated quinolines and 8-quinolinols have been explored,43,44 but the direct use of quinoline moieties as azo photoswitches is rarely reported.
The intercorrelation between azoarene switching motifs and different responsive sites was harnessed for obtaining novel structures and functions.45–48 For example, the attachment of azobenzene onto artificial transporters enabled the fabrication of smart membranes with light-driven transport properties.49–51 Photocontrol over the equilibrium of boronic ester formation and further the stiffness of polymeric hydrogels was achieved with ortho-boronic acid substituted azobenzene.52,53 The incorporation of azobenzene and two dihydroazulene units into a macrocycle allowed the realization of hexa-state switching.54 Moreover, out-of-equilibrium coordination55–58 or dynamic covalent cages59–61 were constructed by making use of azobenzene connected building blocks. A versatile platform for diverse regulation of azoarene switches would be highly desired. While the combination of various switching units and stimuli would build up systematic complexity and enrich chemical information stored in multi-state processes,62–65 it is challenging to develop multi-controllable molecular switching systems with high sophistication and precision. Compared to conventional azobenzene derivatives, azoheteroarenes not only retain the photochromic properties, but also introduce additional basic nitrogen sites that further affect the switching properties through acid–base stimulation, hydrogen bonding, and metal coordination, which would afford ample opportunities for multi-responsive molecular switches.66–70
Along our longstanding efforts in the flourishing field of dynamic covalent chemistry (DCC),71–76 we developed a general concept of dual reactivity based DCC through regulating equilibrating tautomers.77–83 The selection of reaction pathways allowed the creation of switchable reaction networks,78,79 discrete assemblies,80 fluorescent materials,81 as well as dynamic polymers.82,83 Inspired by the controllable switching patterns and abundant signaling outputs, we propose merging azoquinoline photoswitches and ring–chain tautomerism toward the ultimate goal of multi-responsive and multi-state switching systems (Fig. 1a). We envisioned that the incorporation of 2-formylbenzoic acid and quinoline motifs would provide diverse recognition handles, such as acid–base stimulation and metal ion coordination, accordingly influencing ring–chain equilibrium, dynamic covalent reactions (DCRs),84–86 and photoswitching. As a result, the intricate interaction within chemical networks would induce multi-mode responses and enhanced complexity. In the current work multiple control of azoquinoline photoswitches was achieved, including light, pH, metal ions, and amine nucleophiles. The underlying structural and mechanistic foundation was explored, with the interplay between triggers toward modulating switching behaviors in complex systems elucidated and setting the scene for future studies.
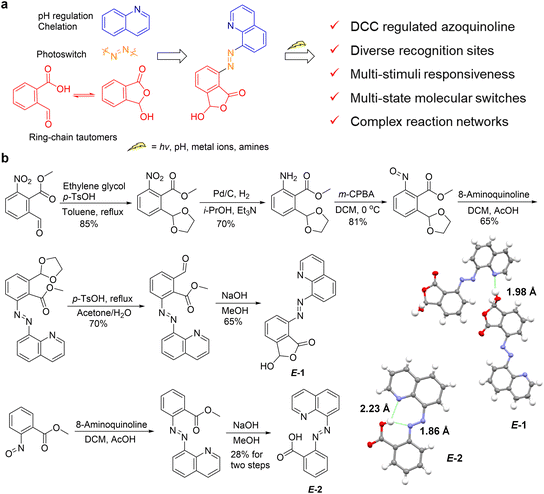 |
| Fig. 1 (a) Proposed strategy for merging azoquinoline photoswitches with ring–chain tautomerism toward the goal of multi-addressed and multi-state switching. (b) Synthesis of target compounds E-1 and E-2 and their corresponding X-ray structures, with the distance (Å) of hydrogen bonding listed. | |
Results and discussion
Design, synthesis, and structures
To realize the strategy a modular synthesis route was developed with 3-nitrophthalic anhydride as the starting material (Scheme S1† and Fig. 1b).87 The ring-opening esterification followed by selective reduction of carboxylic acid and then oxidation of benzylic alcohol afforded methyl 2-nitro-6-formylbenzoate (Scheme S1†). The protection of the aldehyde as a cyclic acetal, reduction of the nitro group, and subsequent nitrosation furnished the key nitroso intermediate (Fig. 1b). The azoarene scaffolds were constructed via azo-coupling reactions from corresponding aromatic amines, and the target compound (E-1) was finally obtained after the hydrolysis of the acetal and the ester groups. A control compound (E-2) without the formyl group was also prepared (Scheme S2†).
With the compounds in place, their solution structures were studied. The appearance of a broad peak around 6.8 ppm suggests that E-1 existed mainly in the cyclic hemiacetal form in DMSO-d6, in equilibrium with the open aldehyde. High-quality single crystals of E-1 were further obtained for XRD analysis (Fig. 1b and S24†). The hemiacetal structure falls in line with solution studies, and quinoline nitrogen orients away from the lactone unit. In addition, both arene groups rotate out of the azo plane (torsion angle around 17°). A supramolecular chain supported by hydrogen bonding between quinoline nitrogen and hydroxyl hydrogen (OH⋯N 1.98 Å) offers additional stabilizing force. In the solid-state structure of E-2, multiple hydrogen bonding between carboxyl OH and two nitrogen atoms (OH⋯N 1.86 and 2.23 Å) was found in a planar structure (Fig. 1b).
Photoswitching behavior
We next set to examine photochromic switching of azoquinoline. The hemiacetal methine hydrogen and the hydrogen next to the quinoline nitrogen were used to determine the ratio of E/Z isomers due to their characteristic chemical shifts. When E-1 (a of Fig. 2A) was subjected to photoisomerization at 365 nm for 1.5 h, the Z isomer (60%) was detected in DMSO-d6, and the cyclic hemiacetal form was maintained, as evidenced by the presence of a broad peak at about 6.9 ppm, partially overlapping with E-1 (b of Fig. 2A). The irradiation at 425 nm allowed the recovery of the E isomer (80%, c of Fig. 2A). Despite the realization of E/Z switching, the thermal stability of Z-1 was modest, with a half-life around 5.4 h (Fig. S25–S27†). Among azoarenes with six-membered heterocycles that have been reported, E-1 has comparable bidirectional switching efficiency and thermal stability,41–44,88–90 but the photoisomerization rate is lower than that of representative azoarenes with five-membered pyrazoles that are nearly quantitatively switchable.29,30 Photoinduced E → Z (82% Z isomer) and Z → E (85% E isomer) isomerization of E-2 in CDCl3 was also attained. The measurement of UV-vis spectra validated E/Z photoswitching, and multiple cycles of bidirectional photoswitching were feasible, demonstrating great reversibility and fatigue-resistance (Fig. 2B and S28, S29†).
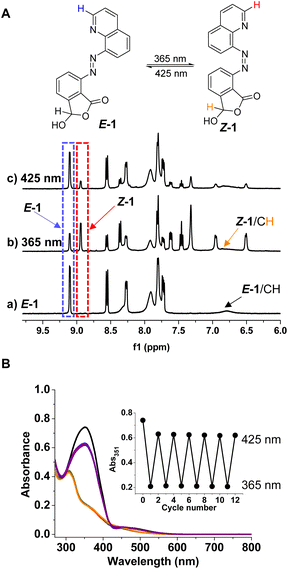 |
| Fig. 2 Photoswitching behavior of E-1. (A) 1H NMR spectra of E-1 in DMSO-d6 (10 mM, a), and after irradiation for 1.5 h at 365 nm (b) and then for 0.5 h at 425 nm (c). (B) UV-vis spectra of E-1 (75 μM in DMSO) after alternative irradiation at 365 and 425 nm. The inset shows the multiple cycles of switching in response to 365 and 425 nm light. | |
pH effects
The regulation of ring–chain tautomerism and photoswitching of the cyclic hemiacetal attached azoquinoline by changing the pH was then explored (Fig. 3A). The addition of methanesulfonic acid (MA) to E-1 gave the protonated form of E-1 (i.e., E-1-H+, Scheme S3†). Both cyclic hemiacetal (6.8 ppm) and open aldehyde (10.2 ppm) forms were observed in DMSO-d6, with a ratio of 5
:
1. XRD analysis (Fig. 3B) verified the lactone form of E-1-H+. Furthermore, an extended planar structure was found, with intramolecular NH⋯N hydroxyl hydrogen bonding (2.43 Å).
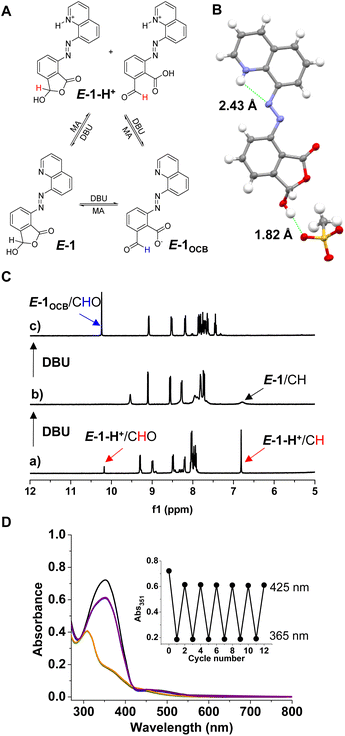 |
| Fig. 3 (A) Controlling the ring–chain tautomerization with acid/base. (B) X-ray structure of E-1-H+, with the distance (Å) of hydrogen bonding listed. (C) 1H NMR spectra of E-1-H+ in DMSO-d6 (10 mM, a), its mixture with DBU (1 equiv., b), and following addition of DBU (1 equiv., c). (D) UV-vis spectra of E-1-H+ (50 μM in DMSO) after alternative irradiation at 365 and 425 nm, with the inset showing the multiple cycles of switching in response to light. | |
The titration of MA into E-1 further confirmed the domination of the ring tautomer for E-1-H+, and the H3 signal of E-1-H+ moved downfield by 0.4 ppm compared to the H3 signal of E-1 at 8.6 ppm (Fig. S30†). The titration of DBU into E-1-H+ (a of Fig. 3C and S31†) gave rise to the deprotonation of quinolinium (i.e., E-1, b of Fig. 3C) followed by ring opening to afford the conjugate base of the open aldehyde (E-1OCB, c of Fig. 3C). The reverse acid-triggered switching from E-1OCB to E-1 and then E-1-H+ was also verified (Fig. S32†). Similar photochromic switching behaviors were also obtained for E-1-H+ in DMSO-d6 (44% Z isomer for E → Z and 87% E isomer for Z → E), albeit with a shorter half-life (1.7 h) for Z-1-H+ over Z-1 (Fig. 3D and S33–S36†), which is likely due to the protonation of the azo group via intramolecular hydrogen transfer from the quinolinium.91,92 Moreover, the cyclic hemiacetal was maintained in Z-1-H+. When the photochromic switching of E-1OCB was investigated in DMSO-d6 (Fig. S37–S41†), it was found that E-1OCB has a relatively high photoisomerization efficiency (69% Z isomer for E → Z) and thermal stability (half-life 20.6 h) over E-1/Z-1. This was attributed to the fact that the use of a base converts E-1 to the ring-opened form E-1OCB, which limits the formation of hydrogen bonds.
Coordination effects
Metal ion coordination was then employed to diversify the switching system (Fig. 4A). Interestingly, the cyclic hemiacetal form (a of Fig. 4B) was converted to an open aldehyde upon the addition of Zn(OAc)2 to E-1, as the methine peak moved from 6.8 to 10.2 ppm (b of Fig. 4B and S42†). Crystal analysis revealed the chelation of zinc with quinoline nitrogen, adjacent azo nitrogen, as well as carboxylate of E-1OCB (Fig. 4C). The additional monodentate coordination by water and the carboxylate from a second molecule of E-1OCB gives a distorted bipyramidal geometry. In order to accommodate metal binding, the carboxyl group rotates out of the arene plane. As compared to E-1-H+, the rotation of one C–N single bond would be needed to facilitate zinc chelation in E-1-Zn2+. The distinct binding mode of a proton and metal ion by E-1 can be attributed to the significantly different size of the guest. Moreover, supramolecular chains of zinc complexes linked by π-stacking and hydrogen bonding stabilize crystal packing. Since carboxylate engages in zinc binding, the adjacent formyl unit would be exposed, resulting in the shift of ring–chain tautomerization equilibrium. With the proton displaced by zinc ion from E-1, acetic acid would also be released. The addition of EDTA enabled the formation of E-1, with additional cycles of complexation/de-complexation feasible (Fig. S43–S45†).
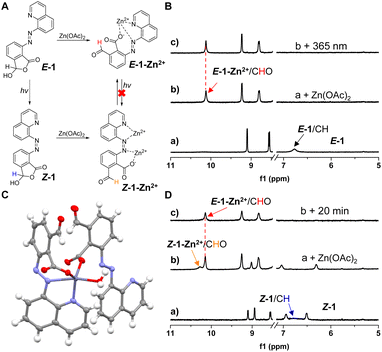 |
| Fig. 4 (A) Illustration of the modulation of E-1 with coordination and light. (B) 1H NMR spectra of E-1 in DMSO-d6 (10 mM, a), its mixture with Zn(OAc)2·2H2O (1.5 equiv., b), and after irradiation for 1.5 h at 365 nm (c). (C) X-ray structure of E-1-Zn2+. (D) 1H NMR spectra of Z-enriched solution of 1 (created by irradiation of E-1 for 1.5 h at 365 nm) in DMSO-d6 (10 mM, a), its mixture with Zn(OAc)2·2H2O (2.5 equiv., b), and after 20 min (c). | |
Furthermore, analogous metal binding induced reversal of ring–chain tautomerism was also realized with Co(OAc)2. The coordination of Co2+ by two molecules of E-1OCB allows the creation of an approximately octahedral configuration according to the crystal structure of E-1-Co2+ (Fig. S24†). In addition, photoswitching was suppressed for E-1-Zn2+ (c of Fig. 4B) and E-1-Co2+ in DMSO-d6 (Fig. S46 and S47†), likely due to the locking of the E isomer in the form of metal chelation. Such a gating phenomenon is consistent with the regulation of E/Z isomerization within azobenzene complexes.93 Nevertheless, when Z-1 was generated first followed by the addition of excess amounts of Zn(OAc)2, a similar open aldehyde form was generated for the zinc complex (Z-1-Zn2+) though it converted back to E-1-Zn2+ after 20 min (Fig. 4D and S48†). This is reasonable because Zn2+ could be chelated by carboxylate and neighboring azo nitrogen and thus induce ring-opening of the lactone (Fig. 4A). Despite the low thermal stability, the otherwise inaccessible high-energy state (E-1-Zn2+) was thus attained through a detour. As a result, the sequence of photoirradiation and coordination further built up the complexity of the switching system.
Dynamic covalent chemistry
The next goal was to tune photoswitching with dynamic covalent reactions (DCRs) of amines in conjunction with metal coordination (Fig. 5A). With the cyclic hemiacetal present in E-1, its reactivity is reminiscent of 2-formylbenzoic acid,84–86 giving open imine E-3 with 1-butylamine (3 equiv.) (Fig. S49†). E-3 showed higher photoisomerization efficiency under 365 nm irradiation (78% Z isomer) than E-1OCB, and no photoisomerization of imines was observed (Fig. S50†).94,95 The Z → E switching at 425 nm (81% E isomer) was also attained. As for the thermal stability, Z-3 exhibited a shorter half-life (9.0 h) than Z-1OCB in DMSO-d6 (Fig. S51†). We speculated that n-butylammonium, created from excess n-butylamine and carboxyl, acts as a guest to bind with Z-3via hydrogen bonding, thus reducing its stability. Therefore, DBU (1 equiv.) was used to capture the protons in n-butylammonium. The half-life was significantly prolonged to 85.6 h, further demonstrating the importance of hydrogen bonding in modulating the thermal stability of azoquinolines (Fig. S52†). Moreover, excellent fatigue-resistance was found when a dilute solution of E-3 was irradiated via alternative illumination at 365 and 425 nm (Fig. S53–S55†). When E-1-Zn2+ (a of Fig. 5B) was subjected to reaction with 1-butylamine (3 equiv.), imine E-3-Zn2+ was afforded (b of Fig. 5B). The ability of photoswitching was turned off for E-3-Zn2+ (c of Fig. 5B and S56†), consistent with E-1-Zn2+. When Z-3 was generated first followed by the addition of Zn(OAc)2, Z-3-Zn2+ appeared and was then converted to E-3-Zn2+ after 30 min (Fig. 5C and S57†). Thus, a complex chemical network was constructed through the intricate interaction with the stimulation of light, metal ions, and amines. The dynamic covalent bonding enables our system to be efficiently post-modified by the imine formation reaction, while the high photoisomerization efficiency after the reaction and abundant recognition sites would show potential applications in smart materials, such as artificial membranes,49 multi-stimuli responsive polymers,96 and nanocapsules.18,20
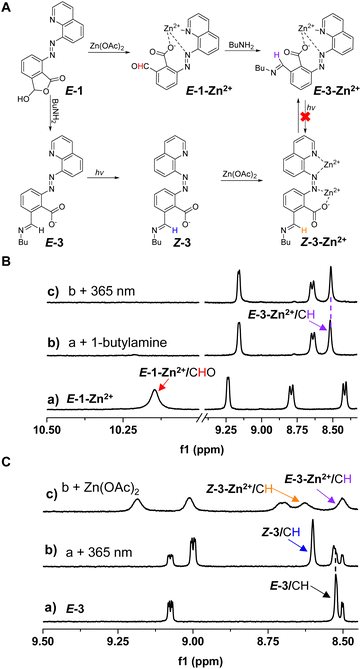 |
| Fig. 5 (A) Illustration of the modulation of E-1 with primary amine, light, and coordination. (B) 1H NMR spectra of E-1-Zn2+ in DMSO-d6 (10 mM, a), its mixture with 1-butylamine (3 equiv., b), and after irradiation for 1.5 h at 365 nm (c). (C) 1H NMR spectra of E-3 in DMSO-d6 (10 mM, a), after irradiation for 1.5 h at 365 nm (b), and its mixture with Zn(OAc)2 (2.5 equiv., c). | |
Reaction networks were further created with secondary amines (Fig. 6A). The reaction of E-1 and piperidine was readily realized, giving cyclic hemiaminal ether E-4 (b of Fig. 6B and S58†). Similar photochromic switching behaviors were obtained for E-4 (60% Z isomer for E → Z and 80% E isomer for Z → E) with multiple cycles of switching successful, but a much shorter half-life (2.3 h) for Z-4 over Z-3 (Fig. S59–S63†). Different from E-3-Zn2+, a combination of E-1-Zn2+ and piperidine resulted in the formation of E-4 and concomitant release of metal ion (b of Fig. 6C and S64†). Moreover, no metal binding was apparent upon the titration of Zn(OAc)2 into E-4 (c of Fig. 6B and S65†). Nevertheless, upon mixing Zn(OTf)2 and E-4 the decomposition of E-4 occurred accompanied by the appearance of E-1-Zn2+, likely due to the higher Lewis acidity of Zn(OTf)2 (c of Fig. 6C and S66†). As a result, the selection of metal salts offered a facile means for control over dynamic covalent reactivity.
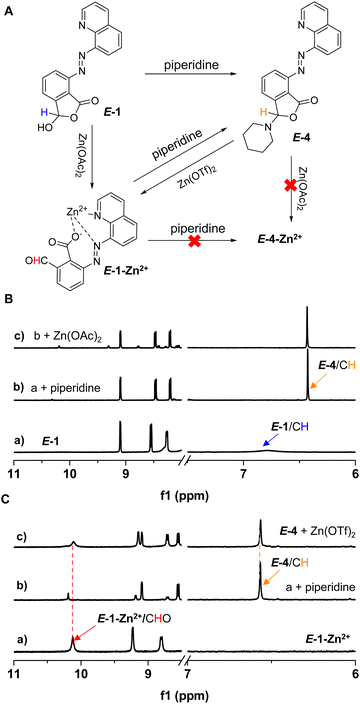 |
| Fig. 6 (A) Illustration of the modulation of E-1 with secondary amine and coordination. (B) 1H NMR spectra of E-1 in DMSO-d6 (10 mM, a), its mixture with piperidine (1.5 equiv., b), and its mixture with Zn(OAc)2 (1.0 equiv., c). (C) 1H NMR spectra of E-1-Zn2+ in DMSO-d6 (10 mM, a), its mixture with piperidine (1.5 equiv., b), as well as the mixture of E-4 and Zn(OTf)2 (3 equiv., c). | |
The discrimination between primary and secondary amines can be attributed to the ring–chain equilibrium of E-3, which renders the carboxyl ligand feasible for engaging in the chelation of Zn2+. In contrast, the cyclic hemiaminal ether for E-4 would give a more rigid scaffold, with zinc binding unlikely. Thus, the distinct metal binding mode of primary and secondary amine derived adducts provided a handle for regulation of the switching behaviors of azoquinolines. Tying it all together, through the interaction between different stimuli we realized multiple control of azoarenes and constructed a multi-state reaction network (Fig. 7).
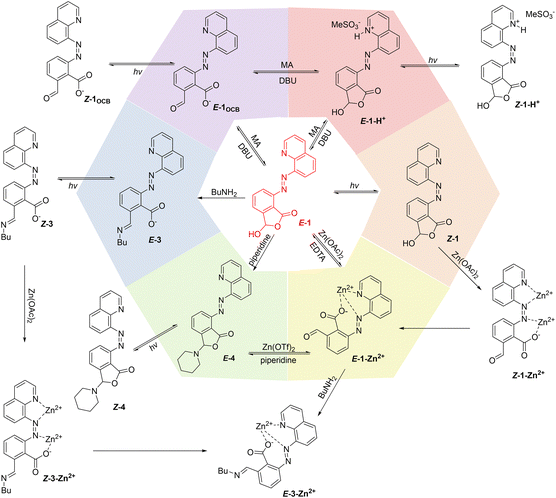 |
| Fig. 7 Illustration of the modulation of E-1 with light, pH, coordination, and amine nucleophiles, and the reaction network created by the interaction between different stimuli. | |
Conclusions
In summary, we developed a multi-responsive molecular switching system through combining an azoquinoline photoswitching unit with ring fusion dynamic covalent sites. The incorporation of a cyclic hemiacetal and quinoline into the azoarene scaffold was realized in a multi-step synthetic sequence. Acid/base stimulation allowed the modulation of ring–chain equilibrium between the cyclic hemiacetal and open aldehyde and E/Z photoswitching behaviors. Furthermore, metal ion chelation by carboxylate as well as azo and quinoline nitrogen was attained, resulting in the reversal of ring–chain equilibrium and turning off the photoswitching. The inaccessible metal ion-containing Z isomer was instead created by changing the stimulation order. Dynamic covalent reactions of primary amines along with light and metal coordination further enhanced the complexity of the system. Differently, reactions with secondary amines prohibit the ring–chain tautomerism and thus complexation. In essence, multiple control of the switching system was achieved with light, pH, metal ions, and amine nucleophiles, with the intricate interaction between different stimuli further affording addressable multi-state switching within the complex network (Fig. 7). This work demonstrates diverse dynamic covalent and non-covalent binding sites as powerful tools to induce abundant triggers in multi-state switching systems, which will open new possibilities in molecular switches, information encoding, and smart materials.
Data availability
All experimental data and detailed experimental procedures are available in the main text and ESI.†
Author contributions
Y. L. carried out the synthetic work, photoswitching studies, and dynamic covalent reactions. H. Y. carried out the theoretical calculation. L. Y. designed the project and wrote the manuscript. All authors discussed the results and have given approval to the final version of the manuscript.
Conflicts of interest
There are no conflicts to declare.
Acknowledgements
We thank NSFC (22071247, 22101283, 22101284, and 92156010), the Strategic Priority Research Program (XDB20000000) and the Key Research Program of Frontier Sciences (QYZDB-SSW-SLH030) of the CAS, NSF of Fujian Province (2020J06035 and 2022J05085), and Fujian Science & Technology Innovation Laboratory for Optoelectronic Information of China (2021ZR112) for funding.
Notes and references
- H. M. D. Bandara and S. C. Burdette, Photoisomerization in different classes of azobenzene, Chem. Soc. Rev., 2012, 41, 1809–1825 RSC.
- H. Chen, W. Chen, Y. Lin, Y. Xie, S. H. Liu and J. Yin, Visible and near-infrared light activated azo dyes, Chin. Chem. Lett., 2021, 32, 2359–2368 CrossRef CAS.
- F. A. Jerca, V. V. Jerca and R. Hoogenboom, Advances and opportunities in the exciting world of azobenzenes, Nat. Rev. Chem, 2022, 6, 51–69 CrossRef PubMed.
- J. Volaric, W. Szymanski, N. A. Simeth and B. L. Feringa, Molecular photoswitches in aqueous environments, Chem. Soc. Rev., 2021, 50, 12377–12449 RSC.
- P. Tecilla and D. Bonifazi, Configurational Selection in Azobenzene-Based Supramolecular Systems Through Dual-Stimuli Processes, ChemistryOpen, 2020, 9, 538–553 CrossRef CAS PubMed.
- A. Blanco-Gómez, P. Cortón, L. Barravecchia, I. Neira, E. Pazos, C. Peinador and M. D. García, Controlled binding of organic guests by stimuli-responsive macrocycles, Chem. Soc. Rev., 2020, 49, 3834–3862 RSC.
- D.-H. Qu, Q. C. Wang, Q.-W. Zhang, X. Ma and H. Tian, Photoresponsive Host-Guest Functional Systems, Chem. Rev., 2015, 115, 7543–7588 CrossRef CAS PubMed.
- C. Nie, C. Liu, S. Sun and S. Wu, Visible-Light-Controlled Azobenzene-Cyclodextrin Host-Guest Interactions for Biomedical Applications and Surface Functionalization, ChemPhotoChem, 2021, 5, 893–901 CrossRef CAS.
- M. Baroncini and G. Bergamini, Azobenzene: A Photoactive Building Block for Supramolecular Architectures, Chem. Rec., 2017, 17, 700–712 CrossRef CAS PubMed.
- D. Dattler, G. Fuks, J. Heiser, E. Moulin, A. Perrot, X. Yao and N. Giuseppone, Design of Collective Motions from Synthetic Molecular Switches, Rotors, and Motors, Chem. Rev., 2020, 120, 310–433 CrossRef CAS PubMed.
- F. Lancia, A. Ryabchun and N. Katsonis, Life-like motion driven by artificial molecular machines, Nat. Rev. Chem, 2019, 3, 536–551 CrossRef CAS.
- M. Baroncini, S. Silvi and A. Credi, Photo- and Redox-Driven Artificial Molecular Motors, Chem. Rev., 2020, 120, 200–268 CrossRef CAS PubMed.
- M. Younis, S. Ahmad, A. Atiq, M. A. Farooq, M.-H. Huang and M. Abbas, Recent Progress in Azobenzene-Based Supramolecular Materials and Applications, Chem. Rec., 2023, 23, e202300126 CrossRef CAS PubMed.
- X. Yao, T. Li, J. Wang, X. Ma and H. Tian, Recent Progress in Photoswitchable Supramolecular Self-Assembling Systems, Adv. Opt. Mater., 2016, 4, 1322–1349 CrossRef CAS.
- X. Cheng, T. Miao, Y. Qian, Z. Zhang, W. Zhang and X. Zhu, Supramolecular Chirality in Azobenzene-Containing Polymer System: Traditional Postpolymerization Self-Assembly Versus In Situ Supramolecular Self-Assembly Strategy, Int. J. Mol. Sci., 2020, 21, 6186 CrossRef CAS PubMed.
- J. Vapaavuori, C. G. Bazuin and A. Priimagi, Supramolecular design principles for efficient photoresponsive polymer-azobenzene complexes, J. Mater. Chem. C, 2018, 6, 2168–2188 RSC.
- J. Krajczewski, R. Ambroziak and A. Kudelski, Photo-assembly of plasmonic nanoparticles: methods and applications, RSC Adv., 2021, 11, 2575–2595 RSC.
- M. Zheng and J. Yuan, Polymeric nanostructures based on azobenzene and their biomedical applications: synthesis, self-assembly and stimuli-responsiveness, Org. Biomol. Chem., 2022, 20, 749–767 RSC.
- T. Bian, Z. Chu and R. Klajn, The Many Ways to Assemble Nanoparticles Using Light, Adv. Mater., 2020, 32, 1905866 CrossRef CAS PubMed.
- H. Che and J. C. M. van Hest, Stimuli-responsive polymersomes and nanoreactors, J. Mater. Chem. B, 2016, 4, 4632–4647 RSC.
- S. Crespi, N. A. Simeth and B. Köinig, Heteroaryl azo dyes as molecular photoswitches, Nat. Rev. Chem, 2019, 3, 133–146 CrossRef CAS.
- H. Ren, P. Yang and F. M. Winnik, Azopyridine: a smart photo- and chemo-responsive substituent for polymers and supramolecular assemblies, Polym. Chem., 2020, 11, 5955–5961 RSC.
- A. Mukherjee, M. D. Seyfried and B. J. Ravoo, Azoheteroarene and Diazocine Molecular Photoswitches: Self-Assembly, Responsive Materials and Photopharmacology, Angew. Chem., Int. Ed., 2023, 62, e202304437 CrossRef CAS PubMed.
- D. Gupta, A. K. Gaur, H. Kumar, S. Singh and S. Venkataramani, Light-Switchable Metal Complexes: Introducing Photoresponsive Behaviour Through Azoheteroarenes, ChemPhotoChem, 2023, 7, e202300068 CrossRef CAS.
- H. Ren, P. Yang and H. Yu, Recent Progress in Azopyridine-Containing Supramolecular Assembly: From Photoresponsive Liquid Crystals to Light-Driven Devices, Molecules, 2022, 27, 3977 CrossRef CAS PubMed.
- P. Gupta, D. P. Karothu, E. Ahmed, P. Naumov and N. K. Nath, Thermally Twistable, Photobendable, Elastically Deformable, and Self-Healable Soft Crystals, Angew. Chem., Int. Ed., 2018, 57, 8498–8502 CrossRef CAS PubMed.
- J. Garcia-Amorós, W. A. Massed, S. Nonell and D. Velasco, Fast Isomerizing Methyl Iodide Azopyridinium Salts for Molecular Switches, Org. Lett., 2010, 12, 3514–3517 CrossRef PubMed.
- J. García-Amorós and D. Velasco, Recent advances towards azobenzene-based light-driven real-time information-transmitting materials, Beilstein J. Org. Chem., 2012, 8, 1003–1017 CrossRef PubMed.
- C. E. Weston, R. D. Richardson, P. R. Haycock, A. J. P. White and M. J. Fuchter, Arylazopyrazoles: Azoheteroarene Photoswitches Offering Quantitative Isomerization and Long Thermal Half-Lives, J. Am. Chem. Soc., 2014, 136, 11878–11881 CrossRef CAS PubMed.
- Y. He, Z. Shangguan, Z.-Y. Zhang, M. Xie, C. Yu and T. Li, Azobispyrazole Family as Photoswitches Combining (Near-) Quantitative Bidirectional Isomerization and Widely Tunable Thermal Half-Lives from Hours to Years, Angew. Chem., Int. Ed., 2021, 60, 16539–16546 CrossRef CAS PubMed.
- A. K. Gaur, D. Gupta, A. Mahadevan, P. Kumar, H. Kumar, D. N. Nampoothiry, N. Kaur, S. K. Thakur, S. Singh, T. Slanina and S. Venkataramani, Bistable Aryl Azopyrazolium Ionic Photoswitches in Water, J. Am. Chem. Soc., 2023, 145, 10584–10594 CrossRef CAS PubMed.
- T. Wendler, C. Schütt, C. Näther and R. Herges, Photoswitchable Azoheterocycles via Coupling of Lithiated Imidazoles with Benzenediazonium Salts, J. Org. Chem., 2012, 77, 3284–3287 CrossRef CAS PubMed.
- T. Yoshida, T. Monji, D. Kawamori, N. Akai, K. Shibuya and A. Kawai, Solvent-dependent Photoisomerization Quantum Yield of 2-Phenylazo-1-alkyl-3-methylimidazolium Cations in Ionic Liquids under S1(n, π*) Excitation, Chem. Lett., 2013, 42, 1490–1492 CrossRef CAS.
- A. Dolai, S. M. Box, S. Bhunia, S. Bera, A. Das and S. Samanta, Photoisomerization of 2-Arylazoimidazoles under Visible Light: Identifying a Predictive Tool to Anticipate and Tune Likely Photoswitching Performance and Cis Half-Life, J. Org. Chem., 2023, 88, 8236–8247 CrossRef CAS PubMed.
- J. Garcia-Amorós, M. C. R. Castro, P. Coelho, M. M. M. Raposo and D. Velasco, New heterocyclic systems to afford microsecond green-light isomerisable azo dyes and their use as fast molecular photochromic switches, Chem. Commun., 2013, 49, 11427–11429 RSC.
- T. Dang, D. Dong, J. Zhang, Y. He, Z.-Y. Zhang and T. Li, Thiazolylazopyrazoles as Nonsymmetric Bis-Heteroaryl Azo Switches: High-Yield Visible-Light Photoisomerization and Increased Z-Isomer Stability by o-Carbonylation, Angew. Chem., Int. Ed., 2023, 62, e202301992 CrossRef CAS PubMed.
- R. Z. Lin, P. K. Hashim, S. Sahu, A. S. Amrutha, N. M. Cheruthu, S. Thazhathethil, K. Takahashi, T. Nakamura, T. Kikukawa and N. Tamaoki, Phenylazothiazoles as Visible-Light Photoswitches, J. Am. Chem. Soc., 2023, 145, 9072–9080 CrossRef CAS PubMed.
- J. Calbo, C. E. Weston, A. J. P. White, H. S. Rzepa, J. Contreras-García and M. J. Fuchter, Tuning Azoheteroarene Photoswitch Performance through Heteroaryl Design, J. Am. Chem. Soc., 2017, 139, 1261–1274 CrossRef CAS PubMed.
- J. Li, H. Hu, T. Jing, C. Zhang, C. Gao, C. Sun, Y. Du and B. Hu, 3-Pyridylazoindole derivatives as photoswitches: Synthesis, photoswitching behavior research, and applied to "on-off" fluorescence sensor for Cu2+ combined with theoretical calculations, J. Photochem. Photobiol., A, 2023, 445, 115059 CrossRef CAS.
- A. Rennhack, E. Grahn, U. B. Kaupp and T. K. Berger, Photocontrol of the Hv1 Proton Channel, ACS Chem. Biol., 2017, 12, 2952–2957 CrossRef CAS PubMed.
- D. Kolarski, W. Szymanski and B. L. Feringa, Two-Step, One-Pot Synthesis of Visible-Light-Responsive 6-Azopurines, Org. Lett., 2017, 19, 5090–5093 CrossRef CAS PubMed.
- L. Cechová, J. Kind, M. Dracínsky, J. Filo, Z. Janeba, C. M. Thiele, M. Cigán and E. Procházková, Photoswitching Behavior of 5-Phenylazopyrimidines: In situ Irradiation NMR and Optical Spectroscopy Combined with Theoretical Methods, J. Org. Chem., 2018, 83, 5986–5998 CrossRef PubMed.
- O. N. Lygo, V. O. Shvydkii, E. N. Khodot, V. A. Ogurtsov, L. N. Kurkovskaya, I. I. Levina and T. D. Nekipelova, Spectral and Time-Resolved Properties of Novel Hetarylazo Dyes Containing Hydrogenated Quinolines and Triazole Moieties, High Energy Chem., 2014, 48, 260–265 CrossRef CAS.
- K. Bujak, A. Wasiak, A. Sobolewska, S. Bartkiewicz, J. G. Malecki, J. E. Nycz, E. Schab-Balcerzak and J. Konieczkowska, A family of azoquinoline derivatives: Effect of the substituent at azo linkage on thermal cis-trans isomerization based on an experimental and computational approach, Dyes Pigm., 2020, 175, 108151 CrossRef CAS.
- P. Kumar, D. Gupta, S. Grewal, A. Srivastava, A. K. Gaur and S. Venkataramani, Multiple Azoarenes Based Systems - Photoswitching, Supramolecular Chemistry and Application Prospects, Chem. Rec., 2022, 22, e202200074 CrossRef CAS PubMed.
- A. Fihey, A. Perrier, W. R. Browne and D. Jacquemin, Multiphotochromic molecular systems, Chem. Soc. Rev., 2015, 44, 3719–3759 RSC.
- S. J. Wezenberg, Photoswitchable molecular tweezers: isomerization to control substrate binding, and what about vice versa?, Chem. Commun., 2022, 58, 11045–11058 RSC.
- D. Zhang, J. Li, H. Xie, A. Zhu, Y. Xu, B. Zeng, W. Luo and L. Dai, Polyion complex micelles formed by azobenzene-based polymer with multi-responsive properties, J. Appl. Polym. Sci., 2021, 138, 50580 CrossRef CAS.
- L. Socrier and C. Steinem, Photo-Lipids: Light-Sensitive Nano-Switches to Control Membrane Properties, ChemPlusChem, 2023, 88, e202300203 CrossRef CAS PubMed.
- J. N. Martins, B. Raimundo, A. Rioboo, Y. Folgar-Cameán, J. Montenegro and N. Basílio, Photoswitchable Calixarene Activators for Controlled Peptide Transport across Lipid Membranes, J. Am. Chem. Soc., 2023, 145, 13126–13133 CrossRef CAS PubMed.
- T. G. Johnson, A. Sadeghi-Kelishadi and M. J. Langton, A Photo-responsive Transmembrane Anion Transporter Relay, J. Am. Chem. Soc., 2022, 144, 10455–10461 CrossRef CAS PubMed.
- J. V. Accardo and J. A. Kalow, Reversibly tuning hydrogel stiffness through photocontrolled dynamic covalent
crosslinks, Chem. Sci., 2018, 9, 5987–5993 RSC.
- J. V. Accardo, E. R. McClure, M. A. Mosquera and J. A. Kalow, Using Visible Light to Tune Boronic Acid-Ester Equilibria, J. Am. Chem. Soc., 2020, 142, 19969–19979 CrossRef CAS PubMed.
- A. Vlasceanu, M. Koerstz, A. B. Skov, K. V. Mikkelsen and M. B. Nielsen, Multistate Photoswitches: Macrocyclic Dihydroazulene/Azobenzene Conjugates, Angew. Chem., Int. Ed., 2018, 57, 6069–6072 CrossRef CAS PubMed.
- R. G. DiNardi, A. O. Douglas, R. Tian, J. R. Price, M. Tajik, W. A. Donald and J. E. Beves, Visible-Light-Responsive Self-Assembled Complexes: Improved Photoswitching Properties by Metal Ion Coordination, Angew. Chem., Int. Ed., 2022, 61, e202205701 CrossRef CAS PubMed.
- A. D. W. Kennedy, R. G. DiNardi, L. L. Fillbrook, W. A. Donald and J. E. Beves, Visible-Light Switching of Metallosupramolecular Assemblies, Chem.–Eur. J., 2022, 28, e202104461 CrossRef CAS PubMed.
- J. Zhu, X. Chen, X. Jin and Q. Wang, Light-driven interconversion of Pd2L4 cage and mononuclear PdL2 mediated by the isomerization of azobenzene ligand, Chin. Chem. Lett., 2023, 34, 108002 CrossRef CAS.
- D. Hugenbusch, M. Lehr, J. S. von Glasenapp, A. J. McConnell and R. Herges, Light-Controlled Destruction and Assembly: Switching between Two Differently Composed Cage-Type Complexes, Angew. Chem., Int. Ed., 2023, 62, e202212571 CrossRef CAS PubMed.
- E. Nieland, J. Voss and B. M. Schmidt, Photoresponsive Supramolecular Cages and Macrocycles, ChemPlusChem, 2023, 88, e202300353 CrossRef CAS PubMed.
- E. Nieland, J. Voss, A. Mix and B. M. Schmidt, Photoresponsive Dissipative Macrocycles Using Visible-Light-Switchable Azobenzenes, Angew. Chem., Int. Ed., 2022, 61, e202212745 CrossRef CAS PubMed.
- M. Ovalle, M. Kathan, R. Toyoda, C. N. Stindt, S. Crespi and B. L. Feringa, Light-Fueled Transformations of a Dynamic Cage-Based Molecular System, Angew. Chem., Int. Ed., 2023, e202214495 CAS.
- X. Su and I. Aprahamian, Hydrazone-based switches, metallo-assemblies and sensors, Chem. Soc. Rev., 2014, 43, 1963–1981 RSC.
- M. Jeong, J. Park and S. Kwon, Molecular Switches and Motors Powered by Orthogonal Stimuli, Eur. J. Org Chem., 2020, 2020, 7254–7283 CrossRef CAS.
- H. Nie, J. L. Self, A. S. Kuenstler, R. C. Hayward and J. R. de Alaniz, Multiaddressable Photochromic Architectures: From Molecules to Materials, Adv. Opt. Mater., 2019, 7, 1900224 CrossRef.
- S.-Z. Pu, Q. Sun, R.-J. Wang, C.-B. Fan and G. Liu, Recent advances in diarylethene-based multi-responsive molecular switches, J. Mater. Chem. C, 2016, 4, 3075–3093 RSC.
- S. Ludwanowski, M. Ari, K. Parison, S. Kalthoum, P. Straub, N. Pompe, S. Weber, M. Walter and A. Walther, pH Tuning of Water-Soluble Arylazopyrazole Photoswitches, Chem.–Eur. J., 2020, 26, 13203–13212 CrossRef CAS PubMed.
- H. Ren, X.-P. Qiu, Y. Shi, P. Yang and F. M. Winnik, pH-Dependent Morphology and Photoresponse of Azopyridine-Terminated Poly(N-isopropylacrylamide) Nanoparticles in Water, Macromolecules, 2019, 52, 2939–2948 CrossRef CAS PubMed.
- J. N. Bull, M. S. Scholz, N. J. A. Coughlan and E. J. Bieske, Isomerisation of an intramolecular hydrogen-bonded photoswitch: protonated azobis(2-imidazole), Phys. Chem. Chem. Phys., 2017, 19, 12776–12783 RSC.
- J. A. Mondal, G. Saha, C. Sinha and D. K. Palit, Photoisomerization dynamics of N-1-methyl-2-(tolylazo) imidazole and the effect of complexation with Cu(II), Phys. Chem. Chem. Phys., 2012, 14, 13027–13034 RSC.
- D. Gupta, A. K. Gaur, D. Chauhan, S. K. Thakur, V. Jeyapalan, S. Singh, G. Rajaraman and S. Venkataramani, Solid-state photochromic arylazopyrazole-based transition metal complexes, Inorg. Chem. Front., 2022, 9, 2315–2327 RSC.
- S. J. Rowan, S. J. Cantrill, G. R. L. Cousins, J. K. M. Sanders and J. F. Stoddart, Dynamic covalent chemistry, Angew. Chem., Int. Ed., 2002, 41, 898–952 CrossRef.
- Y. Jin, C. Yu, R. J. Denman and W. Zhang, Recent advances in dynamic covalent chemistry, Chem. Soc. Rev., 2013, 42, 6634–6654 RSC.
- J. Li, P. Nowak and S. Otto, Dynamic Combinatorial Libraries: From Exploring Molecular Recognition to Systems Chemistry, J. Am. Chem. Soc., 2013, 135, 9222–9239 CrossRef CAS PubMed.
- A. Wilson, G. Gasparini and S. Matile, Functional systems with orthogonal dynamic covalent bonds, Chem. Soc. Rev., 2014, 43, 1948–1962 RSC.
- J. F. Reuther, S. D. Dahlhauser and E. V. Anslyn, Tunable Orthogonal Reversible Covalent (TORC) Bonds: Dynamic Chemical Control over Molecular Assembly, Angew. Chem., Int. Ed., 2019, 58, 74–85 CrossRef CAS PubMed.
- F. Van Lijsebetten, J. O. Holloway, J. M. Winne and F. E. Du Prez, Internal catalysis for dynamic covalent chemistry applications and polymer science, Chem. Soc. Rev., 2020, 49, 8425–8438 RSC.
- L. You, Dual reactivity based dynamic covalent chemistry: mechanisms and applications, Chem. Commun., 2023, 59, 12943–12958 RSC.
- C. Ni, D. Zha, H. Ye, Y. Hai, Y. Zhou, E. V. Anslyn and L. You, Dynamic Covalent Chemistry within Biphenyl Scaffolds: Reversible Covalent Bonding, Control of Selectivity, and Chirality Sensing with a Single System, Angew. Chem., Int. Ed., 2018, 57, 1300–1305 CrossRef CAS PubMed.
- Y. Hai, H. Zou, H. Ye and L. You, Three Switchable Orthogonal Dynamic Covalent Reactions and Complex Networks Based on the Control of Dual Reactivity, J. Org. Chem., 2018, 83, 9858–9869 CrossRef CAS PubMed.
- J. Mao, Y. Hai, H. Ye and L. You, Adaptive Covalent Networks Enabled by Dual Reactivity: The Evolution of Reversible Covalent Bonds, Their Molecular Assemblies, and Guest Recognition, J. Org. Chem., 2020, 85, 5351–5361 CrossRef CAS PubMed.
- H. Zou, Y. Hai, H. Ye and L. You, Dynamic Covalent Switches and Communicating Networks for Tunable Multicolor Luminescent Systems and Vapor-Responsive Materials, J. Am. Chem. Soc., 2019, 141, 16344–16353 CrossRef CAS PubMed.
- Y. Hai, H. Ye, Z. Li, H. Zou, H. Lu and L. You, Light-Induced Formation/Scission of C-N, C-O, and C-S Bonds Enables Switchable Stability/Degradability in Covalent Systems, J. Am. Chem. Soc., 2021, 143, 20368–20376 CrossRef CAS PubMed.
- H. Chen, C. Cui, H. Ye, H. Zou and L. You, Enhancing hydrolytic stability of dynamic imine bonds and polymers in acidic media with internal protecting groups, Chin. Chem. Lett., 2023 DOI:10.1016/j.cclet.2023.109145.
- P. Kovarícek and J. M. Lehn, Directional Dynamic Covalent Motion of a Carbonyl Walker on a Polyamine Track, Chem.–Eur. J., 2015, 21, 9380–9384 CrossRef PubMed.
- P. Kovarícek, A. C. Meister, K. Flidrová, R. Cabot, K. Kovarícková and J. M. Lehn, Competition-driven selection in covalent dynamic networks and implementation in organic reactional selectivity, Chem. Sci., 2016, 7, 3215–3226 RSC.
- Z. Li, L. Zhang, Y. Zhou, D. Zha, Y. Hai and L. You, Dynamic Covalent Reactions Controlled by Ring-Chain Tautomerism of 2-Formylbenzoic Acid, Eur. J. Org Chem., 2022, 2022, e202101461 CrossRef CAS.
- H. Zhang, S. Zhang, L. Liu, G. Luo, W. Duan and W. Wang, Synthesis of Chiral 3-Substituted Phthalides by a Sequential Organocatalytic Enantioselective
Aldol-Lactonization Reaction. Three-Step Synthesis of (S)-(-)-3-Butylphthalide, J. Org. Chem., 2010, 75, 368–374 CrossRef CAS PubMed.
- J. Garcia-Amorós, S. Nonell and D. Velasco, Light-controlled real time information transmitting systems based on nanosecond thermally-isomerising amino-azopyridinium salts, Chem. Commun., 2012, 48, 3421–3423 RSC.
- S. Thies, H. Sell, C. Bornholdt, C. Schütt, F. Köhler, F. Tuczek and R. Herges, Light-Driven Coordination-Induced Spin-State Switching: Rational Design of Photodissociable Ligands, Chem.–Eur. J., 2012, 18, 16358–16368 CrossRef CAS PubMed.
- M. Nakagawa, M. Rikukawa, M. Watanabe, K. Sanui and N. Ogata, Photochromic, electrochemical, and photoelectrochemical properties of novel azopyridinium derivatives, Bull. Chem. Soc. Jpn., 1997, 70, 737–744 CrossRef CAS.
- S. Samanta, A. Babalhavaeji, M.-X. Dong and G. A. Woolley, Photoswitching of ortho-Substituted Azonium Ions by Red Light in Whole Blood, Angew. Chem., Int. Ed., 2013, 52, 14127–14130 CrossRef CAS PubMed.
- N. A. Simeth, S. Crespi, M. Fagnoni and B. König, Tuning the Thermal Isomerization of Phenylazoindole Photoswitches from Days to Nanoseconds, J. Am. Chem. Soc., 2018, 140, 2940–2946 CrossRef CAS PubMed.
- R. Tanaka, P. Viehmann and S. Hecht, Bis(phenoxy-azo)titanium(IV) Complexes: Synthesis, Structure, and Catalytic Activity in Styrene Polymerization, Organometallics, 2012, 31, 4216–4220 CrossRef CAS.
- L. Greb, A. Eichhöfer and J. M. Lehn, Synthetic Molecular Motors: Thermal N Inversion and Directional Photoinduced C=N Bond Rotation of Camphorquinone Imines, Angew. Chem., Int. Ed., 2015, 54, 14345–14348 CrossRef CAS PubMed.
- L. Greb and J. M. Lehn, Light-Driven Molecular Motors: lmines as Four-Step or Two-Step Unidirectional Rotors, J. Am. Chem. Soc., 2014, 136, 13114–13117 CrossRef CAS PubMed.
- X. Zheng, Q. Bian, C. Ye and G. Wang, Visible light-, pH-, and cyclodextrin-responsive azobenzene functionalized polymeric nanoparticles, Dyes Pigm., 2019, 162, 599–605 CrossRef CAS.
Footnote |
† Electronic supplementary information (ESI) available: Experimental details, X-ray data, NMR, UV-vis, and mass spectra. CCDC 2305271–2305275. For ESI and crystallographic data in CIF or other electronic format see DOI: https://doi.org/10.1039/d3sc05879d |
|
This journal is © The Royal Society of Chemistry 2024 |
Click here to see how this site uses Cookies. View our privacy policy here.