DOI:
10.1039/D4SC00500G
(Edge Article)
Chem. Sci., 2024,
15, 5333-5339
Azasulfur(IV) derivatives of sulfite and sulfinate esters by formal S–S bond insertion of dichloramines†
Received
21st January 2024
, Accepted 4th March 2024
First published on 5th March 2024
Abstract
Azasulfur(VI) compounds such as sulfoximines and sulfonimidamides are attractive due to the unique properties of the S
N bond. While the synthesis of these carbon-attached sulfonimidoyl derivatives is well-established, the situation is different for their heteroatom-bound counterparts. In this work, we propose azasulfur(IV) esters as platform chemicals that can be derivatized to obtain all types of SVI
N functional groups, among these are the poorly accessible, all-heteroatom imidosulfate esters. Using a chloroamination workflow established here, S–S bond-containing structures such as elemental sulfur or diaryl disulfides can be transformed into imidothionyl or sulfinimidoyl chlorides, which are easily esterified or amidated. Thus, chloramines serve as a versatile [N] and [Cl+] source, and by using them in the context reported here, we advance the set of mild synthetic methods as the latest toolbox member to cover even more of the azasulfur(IV) and (VI) chemical space.
Introduction
Azasulfur(VI) structures, with their tetrahedral geometry and formal S
N double bond, represent a class of sulfur compounds that is gaining increasing attention.1 While they can be considered as variations of the more common sulfonyl unit, the nitrogen atom in the sulfonimidoyl core represents an additional point of possible functionalization and imparts considerable physicochemical differences due to its relative basicity and H-bonding ability.2 Combined with good hydrolytic stability, azasulfur(VI) groups such as sulfoximines or sulfonimidamides are increasingly investigated as viable options in medicinal chemistry (Scheme 1A).2a,3
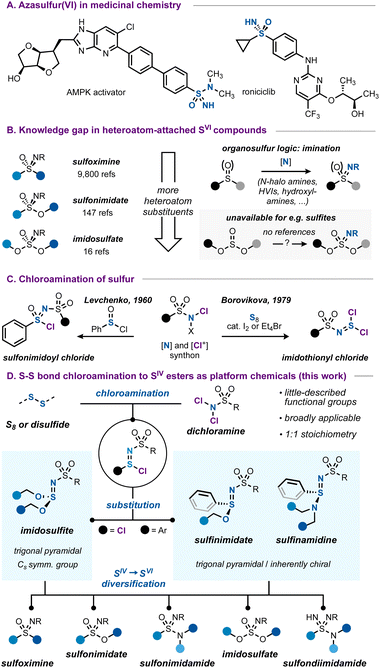 |
| Scheme 1 (A) Azasulfur-containing bioactive molecules. (B) Connection between the carbon-bound azasulfur(VI) literature and all-heteroatom azasulfur(VI) species. (C) Precedents in the literature on the chloroamination of sulfur to form S N bonds. (D) This work. | |
While carbon-bound azasulfur(VI) structures such as sulfoximines are synthetically well-studied, the case changes when more heteroatoms are introduced. For example, considering the sulfonimidate functional group, the number of references is almost seventy-fold smaller compared to those on the topic of sulfoximines, decreasing even further for all-heteroatom imidosulfates (Scheme 1B).4 The number of synthetic entries to these imidosulfur species is accordingly much smaller, and the typical ‘imination’ routes1d,f that work for sulfides or sulfoxides (e.g. transition metal-catalyzed5 or uncatalyzed processes with hydroxylamines,6 hypervalent iodinanes7 or N-halo amides8 as the nitrogen source) are much less translatable to the case of heteroatom-substituted substrates such as sulfinate or sulfite esters.9,10
A complement to this gap in the imination toolbox is therefore needed, enabling a broad access to azasulfur derivatives with non-carbon substituents. The chloroamination reaction of S–S containing species poses a solution to this lack of synthetic routes.
The chloroamination approach to azasulfur preparation finds precedence in the work of Levchenko, who used chloramine T to prepare sulfonimidoyl chlorides (Scheme 1C).11 Later, the same authors reported that chloroamination of elemental sulfur led to imidothionyl chlorides, reactive azasulfur(IV) chemicals with bis(electrophile) potential.12 Imidothionyl halides have been known since the 1960s13 and important work by Shreeve,14 Tisnes,15 Mews,16 and especially by many scientists at the Ukrainian N.A.S.12,17 has greatly expanded the known realm of azasulfur(IV) mono- and dihalides and their applications. Intriguingly, these studies have largely remained limited to the synthesis of SIV compounds, and it seems that little investigation has been made into their usage as general routes to azasulfur(VI) compounds.18
In this work, we aim to develop a broadly applicable chloroamination of sulfur and disulfides, as a general means to obtain heteroatom-attached azasulfur compounds. We reasoned that dichloramines, as stable and often crystalline N–Cl compounds, can serve ideally as an integrated [Cl+] and nitrogen source, capable of being inserted by low-oxidation-state sulfur species.19 The resulting sulfur(IV) chlorides with the ‘sulfinimidoyl’ core can then be transformed into imidosulfites,14a,b,15,17b sulfinimidates6a,20 or sulfinamidines.6a,8d,20d,21 Such compounds are stable, but little-described, and their facile preparation from commercial starting materials contrasts the typical routes to, for example, sulfinimidates, which commonly start from pre-synthesized sulfenamides or sulfinylamines.22 A variety of these S
N derivatives is prepared to explore the synthetic breadth of these functional groups. Ultimately, we demonstrate how azasulfur(IV) compounds can serve as a strategic choice for platform chemicals, enabling access to all classes of heteroatom-attached azasulfur(VI) species.
Results and discussion
We started by focusing our attention on imidothionyl chlorides. Reasoning that these structures are isoelectronic to thionyl chloride, we surmised that they could serve as precursors to imidosulfite esters, which in turn could be transformed into downstream SVI all-heteroatom derivatives. To this end, we set out to optimize the chloroamination of elemental sulfur using a commercial dichloroamine, N,N-dichlorotoluenesulfonamide (dichloramine T, 1).12 By trapping the formed tosylimidothionyl chloride with the preformed disodium salt of neopentylene glycol, various conditions were tested to attain the highest amount of the ester product 3v (Table 1). It was found that the TBAB-catalyzed reaction between TsNCl2 and elemental sulfur in a 1
:
1 ratio in 1,2-DCE was ideal, resulting in an initial 76% yield of 3v after 1 h (Table 1, entry 1). Other chlorinated or aromatic solvents (Table 1, entry 2) or phase-transfer catalysts (Table 1, entry 3) as well as a shortening of the reaction times to only 5 min proved less effective (Table 1, entry 4). Desiccants did not improve the yield (Table 1, entry 5). In the absence of light, however, the yield of 3v increased to 81%. The same outcome resulted when the diol was simply added to the imidothionyl chloride mixture along with Et3N in the same solvent (Table 1, entry 7). The latter conditions constitute the final, optimized conditions.
Table 1 Optimization of the chloroamination of elemental sulfur and subsequent esterification
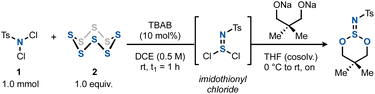
|
Entry |
Deviation from standard conditions |
Yield of 3va (%) |
1H NMR yield relative to the internal standard.
After chromatography.
|
1 |
None |
76 |
2 |
Toluene or CHCl3 as solvent |
52–69 |
3 |
Other Br− or I− catalysts |
<63 |
4 |
t
1 = 5 min |
63 |
5 |
Added desiccants |
<43 |
6 |
In the dark |
81 |
7 |
Diol (1.1 equiv.) + Et3N (2.2 equiv.) in DCE for 30 min |
81
|
The optimal two-step one-pot method was then investigated as a general route to various imidosulfites (Scheme 2, products 3) and imidosulfate esters (products 4). The latter S(VI) compounds were obtained cleanly by a RuCl3/NaIO4-mediated oxidation step.23,24 Being inspired by Fox's work, where fluoroalkyl sulfite esters had shown a remarkable stability,25,26 the investigation of the alcohol scope of the reaction focused initially on halogenated alcohols. Indeed, polyhalogenated alcohols gave good yields of the imidosulfites 3a–h, which underwent the subsequent oxidation efficiently in most cases. In comparison, methanol, ethanol and isopropanol gave mediocre yields of products 3i–k. tert-Butanol did not react.27 We assume that the enhanced stability of polyhalogenated alkyl (and by extension, neopentyl) SIV esters is due to an increased hydrophobicity, greater steric shielding of the sulfur core, the absence of β-protons, or an interplay of these factors. A side note should be added on the oxidation of less halogenated alkyl imidosulfite esters (3c, 3d, 3i, and 3j), where the corresponding imidosulfates were not formed. Instead, they gave rise to different oxidation products, which we believe to be the respective N-tosyl-N,O-dialkylsulfamates (see ESI Sections 2.11 and 2.12†).28 It is conceivable that the corresponding imidosulfate oxidation products 4 were formed initially, but, being good alkylating agents (comparable to, for example, Me2SO4), they became engaged in a rearrangement to form isomeric N-alkylated sulfamates. A similar O-to-N transfer has been reported for O-alkylsulfonimidates by Maricich.29 Interestingly, the other imidosulfites, bearing alkyl groups with a higher degree of halogenation, did not show such side reactivity. In addition, for bulky 3e and 3k neither the desired products 4 nor the rearranged side-products were obtained.
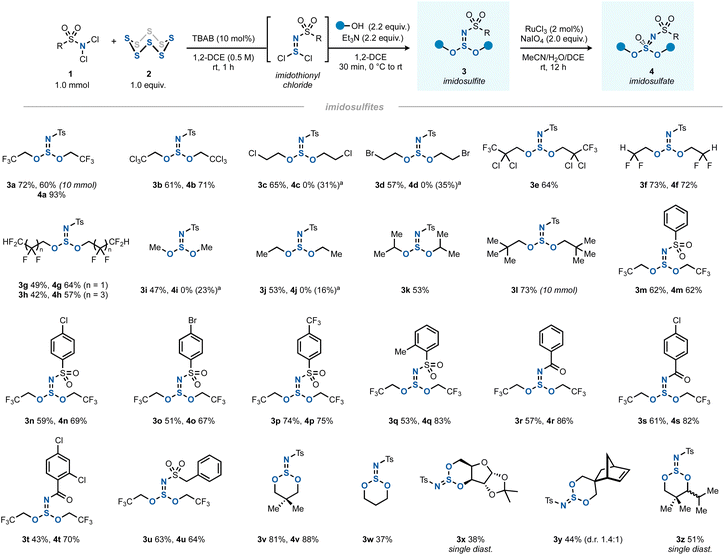 |
| Scheme 2 The scope of synthesis of imidosulfites 3 (1.0 mmol scale) and imidosulfates 4 (0.2 mmol scale). aThe desired imidosulfate was not observed. Instead, N-tosyl-N,O-dialkylsulfamate was isolated (yield between parentheses), see ESI Sections 2.11 and 2.12† for details. | |
Moving away from N-Ts, several other arenesulfonyl (3m–q) and benzoyl (3r–t) protecting groups as well as an aliphatic sulfonyl group (3u) worked well. In all cases, the oxidation yields were good (4m–u). Several cyclic derivatives were prepared by using 1,3-diols as bis(nucleophiles) (3v–z). Five-membered cyclic sulfites (e.g. from ethylene glycol) could not be isolated, possibly due to their well-documented hydrolytic instability.30
Due to the trigonal pyramidal geometry of the imidosulfite moiety, the sulfur atom acts as a rigid stereocenter in the six-membered ring. Derivatives with a distal carbon stereocenter such as 3y therefore gave rise to a mixture of diastereomers. When the stereogenic centers were closer together, a single diastereomer was obtained (3x and 3z). The case of 3z indicates that the iPr-attached carbon stereocenter most likely dictates the orientation of the azasulfur bond. While usually a cis-1,3-disubstituted cyclohexane with both substituents in the equatorial position is the more favourable geometry, a different situation was found for cyclic imidosulfites. As previously described by Tisnes, the anomeric effect (lone pair–lone pair repulsion between heteroatoms) favors the imido nitrogen in the axial position of the ring.15a Indeed, calculations on the DFT level (B3LYP/def2-TZVPP//B3LYP/def2-SVP) indicated a 3.7 kcal mol−1 free energy benefit for trans-3z relative to cis-3z, which was roughly between the values for the steric strain that the iPr and tBu groups experience in the axial position of monosubstituted cyclohexanes.31
Having thoroughly investigated the imidothionyl chloride synthesis by a formal chloroamination of elemental sulfur, we hypothesized that this chemistry could be extended to other types of S–S bonds. Indeed, when using a diaryl disulfide as the sulfur source instead of S8 and a 2
:
1 ratio of dichloramine 1 to disulfide 5, a clean conversion to the corresponding sulfinimidoyl chloride was found (Scheme 3).32 Interestingly, this reaction proceeded catalyst-free without a diminished yield.
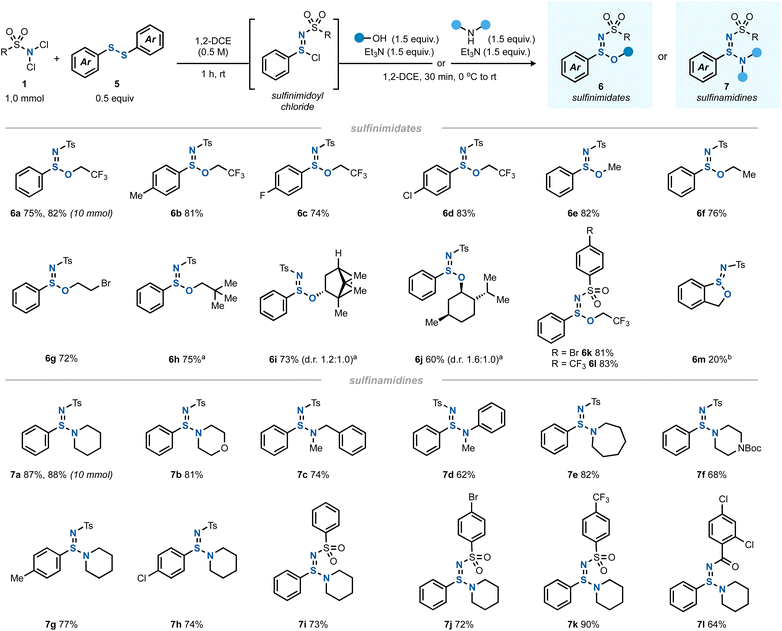 |
| Scheme 3 The scope of synthesis of sulfinimidates 6 (1.0 mmol scale) and sulfinamidines 7 (1.0 mmol scale). aUse of 2.2 equiv. of alcohol and 2.2 equiv. of Et3N instead of the indicated quantities. bUse of 2-mercaptobenzyl alcohol as both the sulfur source and alcohol. See the ESI† for all details. | |
The formed sulfinimidoyl chlorides could be reacted – in analogy to imidothionyl chlorides – with an alcohol/Et3N mixture to give sulfinimidate esters 6 (Scheme 3). With trifluoroethanol, various diaryl disulfides gave rise to the desired products 6a–d. A limitation was found for dialkyl disulfides (see ESI Section 2.13†).
Other primary alcohols afforded the corresponding sulfinimidate esters (6e–h) in good yields, too. Interestingly, halogenated and other aliphatic alcohols reacted equally well and the yields of the resulting sulfinimidate esters 6 were comparable. The chiral secondary alcohols (+)-borneol (6i) and (−)-menthol (6j) led to nonequal mixtures of diastereomers, indicating a stereoinduction to some degree by the existing chiral center. Other N,N-dichlorosulfonamides underwent the reaction equally well to give 6k and 6l. In an intramolecular variant, 2-mercaptobenzyl alcohol was cyclized to give benzoxathiolane 6m albeit in only 20% yield.
Apart from alcohols, amines also served as capable nucleophiles to trap the in situ generated sulfinimidoyl chlorides giving sulfinamidines 7 (Scheme 3). A variety of secondary amines underwent the reaction in good yields, including cyclic (to give 7a, 7b, 7e, and 7f), acyclic (leading to 7c) and aromatic amines (providing 7d). The variation of the disulfide or dichloramine reaction partners posed no problem and sulfinamidines 7g and 7h as well as 7i–l were obtained in good yields.
The next goal was to apply the SIV esters and amines formed by the chloroamination method to the preparation of complex azasulfur(VI) derivatives (Scheme 4). Sulfinimidate 6a was taken as a platform to explore various post-transformations, such as the C–S bond forming substitution reaction with EtMgBr to give arylalkyl sulfilimine 8,20c which could then be oxidized by a known Ru-catalyzed protocol to sulfoximine 9.23 The reaction of 6a with 4-tert-butylphenylmagnesium bromide gave rise to diaryl sulfilimine 10. The direct oxidation of 6a yielded sulfonimidate 11, and a sonochemical method that is known for the transamidation of sulfinate esters was successful for the preparation of 7a.33 In an alternative derivatization, the free sulfinamidine 13 could be obtained from the reaction of 6a with LiHMDS.34 Applying 13 in a subsequent S–N bond forming reaction using Willis' PhI(OAc)2-mediated synthesis gave sulfondiimidamide 14.35 In addition, also imidosulfite ester 3b could undergo (mono)substitution, giving imidosulfamite 15 after reaction with piperidine. An analogous reaction between 3b and PhONa remained unsuccessful.
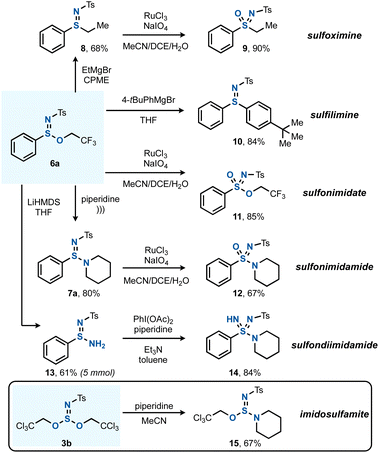 |
| Scheme 4 Diversification to various SIV and SVI functionalities. See the ESI† for full experimental details. | |
Conclusions
In conclusion, this work details a general workflow for synthesizing N-protected azasulfur(IV) esters and amides. From a lack of routes to heteroatom-attached structures with a ‘sulfinimidoyl’ (S
NR) core, the chloroamination of S–S bonds was established to address the need for a general entry to these underdeveloped compound classes. The reactive intermediates formed, either imidothionyl or sulfinimidoyl chlorides, function as versatile electrophiles that could be efficiently trapped with alcohols or amines as nucleophiles. A special role is withheld for halogenated alcohols, which impart high stability to the class of imidosulfite esters. This method has the advantages of using an ideal stoichiometry between commercial sulfur sources (elemental S or disulfides), and stable dichloramines as both oxidant and nitrogen sources. Importantly, the azasulfur(IV) derivatives obtained here are shown to be strategic platform chemicals to a wide variety of azasulfur(VI) compounds, further enabling the increasing trend toward their investigation as novel pharmacophores.
Data availability
Raw NMR data are available from the corresponding author upon request.
Author contributions
J. D. designed the study, P. W. and J. D. conducted the experiments on the synthesis of starting materials, optimization of conditions, substrate exploration, and product transformation. B. J. S. performed the DFT NMR calculations. J. D. wrote the initial version of the manuscript, and P. W. drafted the ESI.† C. B. directed the project and prepared the final draft of the manuscript. All authors have given approval to the final version of the manuscript.
Conflicts of interest
The authors declare no conflict of interest.
Acknowledgements
We are indebted to RWTH Aachen University for generous research funding and infrastructure. KU Leuven and, in particular, Prof. Wim De Borggraeve are thanked for the possibility to use the infrastructure and analytical facilities. Dr Christoph Räuber (RWTH Aachen University) and Dr Gert Steurs (KU Leuven) are kindly acknowledged for assistance with NMR measurements. Simulations were performed with computing resources granted by RWTH Aachen University under project rwth1465. P. W. thanks the CSC (China Scholarship Council) for a predoctoral stipend. J. D. acknowledges Research Foundation – Flanders (FWO) for funding received through fellowships and travel grants (12ZL820N and V433620N). Mass spectrometry was made possible by the support of the Hercules Foundation of the Flemish Government (grant 20100225-7). This research was supported by the Research Foundation Flanders (FWO) through infrastructure grants I002720N and I001920N.
Notes and references
- For recent overviews and paper on sulfoximines see:
(a) P. Mäder and L. Kattner, J. Med. Chem., 2020, 63, 14243–14275 CrossRef PubMed;
(b) M. Andresini, A. Tota, L. Degennaro, J. A. Bull and R. Luisi, Chem.–Eur. J., 2021, 27, 17293–17321 CrossRef CAS PubMed;
(c) V. Bizet, C. M. M. Hendriks and C. Bolm, Chem. Soc. Rev., 2015, 44, 3378–3390 RSC;
(d) D. Zeng, Y. Ma, W.-P. Deng, M. Wang and X. Jiang, Nat. Synth., 2022, 1, 455–463 CrossRef;
(e) U. Lücking, Org. Chem. Front., 2019, 6, 1319–1324 RSC ; for recent overview and paper on sulfonimidamides see:;
(f) G. C. Nandi and P. I. Arvidsson, Adv. Synth. Catal., 2018, 360, 2976–3001 CrossRef CAS;
(g) S. V. Zasukha, V. M. Timoshenko, A. A. Tolmachev, V. O. Pivnytska, O. Gavrylenko, S. Zhersh, Y. Shermolovich and O. O. Grygorenko, Chem.–Eur. J., 2019, 25, 6928–6940 CrossRef CAS PubMed ; for a recent overview on sulfondiimines see:;
(h) M. T. Passia, J. H. Schöbel and C. Bolm, Chem. Soc. Rev., 2022, 51, 4890–4901 RSC ; for a recent paper on sulfondiimidamides see:;
(i) Z.-X. Zhang and M. C. Willis, Chem, 2022, 8, 1137–1146 CrossRef CAS ; for a recent overview on the synthesis and application of azasulfur(VI) compounds via SuFEx linkers see: ;
(j) D. Zeng, W.-P. Deng and X. Jiang, Natl. Sci. Rev., 2023, 10, nwad123 CrossRef CAS PubMed.
-
(a) P. K. Chinthakindi, T. Naicker, N. Thota, T. Govender, H. G. Kruger and P. I. Arvidsson, Angew. Chem., Int. Ed., 2017, 56, 4100–4109 CrossRef CAS PubMed;
(b) M. Frings, C. Bolm, A. Blum and C. Gnamm, Eur. J. Med. Chem., 2017, 126, 225–245 CrossRef CAS PubMed.
-
(a) F. Izzo, M. Schäfer, P. Lienau, U. Ganzer, R. Stockman and U. Lücking, Chem.–Eur. J., 2018, 24, 9295–9304 CrossRef CAS PubMed;
(b) J. A. Sirvent and U. Lücking, ChemMedChem, 2017, 12, 487–501 CrossRef CAS PubMed;
(c) U. Lücking, Angew. Chem., Int. Ed., 2013, 52, 9399–9408 CrossRef PubMed;
(d) For a general view with several examples from sulfur chemistry, see: N. A. Meanwell, J. Agric. Food Chem., 2023, 71, 18087–18122 CrossRef CAS PubMed.
-
Based on a SciFinder search, 2023 Search PubMed.
- O. García Mancheño and C. Bolm, Chem.–Eur. J., 2007, 13, 6674–6681 CrossRef PubMed.
-
(a) M. Andresini, M. Spennacchio, G. Romanazzi, F. Ciriaco, G. Clarkson, L. Degennaro and R. Luisi, Org. Lett., 2020, 22, 7129–7134 CrossRef CAS PubMed;
(b) V. Bizet, L. Buglioni and C. Bolm, Angew. Chem., Int. Ed., 2014, 53, 5639–5642 CrossRef CAS PubMed;
(c) C. S. Tomooka, D. D. LeCloux, H. Sasaki and E. M. Carreira, Org. Lett., 1999, 1, 149–152 CrossRef CAS PubMed;
(d) W. Franek and P. K. Claus, Monatsh. Chem., 1990, 121, 539–547 CrossRef CAS;
(e) H. Lebel, H. Piras and J. Bartholoméüs, Angew. Chem., Int. Ed., 2014, 53, 7300–7304 CrossRef CAS PubMed;
(f) S. Chatterjee, S. Makai and B. Morandi, Angew. Chem., Int. Ed., 2021, 60, 758–765 CrossRef CAS PubMed;
(g) H. Yu, Z. Li and C. Bolm, Angew. Chem., Int. Ed., 2018, 57, 324–327 CrossRef CAS PubMed.
-
(a) R. Luisi and J. A. Bull, Molecules, 2023, 28, 1120–1134 CrossRef CAS PubMed;
(b) J. F. K. Müller and P. Vogt, Tetrahedron Lett., 1998, 39, 4805–4806 CrossRef;
(c) M. Zenzola, R. Doran, L. Degennaro, R. Luisi and J. A. Bull, Angew. Chem., Int. Ed., 2016, 55, 7203–7207 CrossRef CAS PubMed;
(d) D. Leca, K. Song, M. Amatore, L. Fensterbank, E. Lacôte and M. Malacria, Chem.–Eur. J., 2004, 10, 906–916 CrossRef CAS PubMed.
-
(a) A. L. Marzinzik and K. B. Sharpless, J. Org. Chem., 2001, 66, 594–596 CrossRef CAS PubMed;
(b) R. W. Heintzelman and D. Swern, Synthesis, 1976, 731–733 CrossRef CAS;
(c) O. García Mancheño, O. Bistri and C. Bolm, Org. Lett., 2007, 9, 3809–3811 CrossRef PubMed;
(d) G.-F. Yang, H.-S. Huang, X.-K. Nie, S.-Q. Zhang, X. Cui, Z. Tang and G.-X. Li, J. Org. Chem., 2023, 88, 4581–4591 CrossRef CAS PubMed.
- Two examples were found for imination reactions on sulfinate esters as starting materials (see ref. 10) and no examples were found for organic sulfites.
-
(a) A. Tota, S. St John-Campbell, E. L. Briggs, G. O. Estévez, M. Afonso, L. Degennaro, R. Luisi and J. A. Bull, Org. Lett., 2018, 20, 2599–2602 CrossRef CAS PubMed;
(b) X. Zhang, E. C. X. Ang, Z. Yang, C. W. Kee and C.-H. Tan, Nature, 2022, 604, 298–303 CrossRef CAS PubMed.
- E. S. Levchenko, N. Y. Derkach and A. V. Kirsanov, Zh. Obshch. Khim., 1960, 30, 1971–1975 CAS.
- G. S. Borovikova, E. S. Levchenko and E. I. Borovik, Zh. Org. Khim., 1979, 15, 2485–2490 CAS.
- W. C. Smith, C. W. Tullock, R. D. Smith and V. A. Engelhardt, J. Am. Chem. Soc., 1960, 82, 551–555 CrossRef CAS.
-
(a) T. Abe and J. M. Shreeve, Inorg. Chem., 1980, 19, 3063–3067 CrossRef CAS;
(b) O. Glemser and J. M. Shreeve, Inorg. Chem., 1979, 18, 213–214 CrossRef CAS;
(c) J. M. Shreeve, Isr. J. Chem., 1978, 17, 1–10 CrossRef CAS.
-
(a) P. Tisnes, C. Picard, J. D. Bastide, C. Zedde, L. Cazaux, P. Maroni and G. Trinquier, Spectrochim. Acta, Part A, 1983, 39, 943–953 CrossRef;
(b) C. Picard, L. Cazaux and P. Tisnes, Phosphorus, Sulfur, Silicon Relat. Elem., 1981, 10, 35–41 CrossRef CAS.
- R. Mews and O. Glemser, Inorg. Chem., 1972, 11, 2521–2525 CrossRef CAS.
-
(a) E. S. Levchenko and I. E. Sheinkman, Zh. Obshch. Khim., 1966, 36, 428–432 CAS;
(b) L. N. Markovskii, G. S. Fedyuk and E. S. Levchenko, Zh. Org. Khim., 1970, 6, 2426–2431 CAS;
(c) L. N. Markovskii, G. S. Fedyok and Y. G. Bal'On, Zh. Org. Khim., 1974, 10, 1434–1438 CAS;
(d) G. S. Borovikova, E. S. Levchenko and E. M. Dorokhova, Zh. Org. Khim., 1979, 15, 479–485 CAS;
(e) L. N. Markovskii, G. S. Fedyuk, E. S. Levchenko and A. V. Kirsanov, Zh. Org. Khim., 1973, 9, 2502–2506 CAS;
(f) L. N. Markovskii, T. N. Dubinina, E. S. Levchenko and V. V. Pen'kovskii, Zh. Org. Khim., 1976, 12, 1049–1054 CAS;
(g) E. S. Levchenko, L. V. Budnik and T. N. Dubinina, Zh. Org. Khim., 1978, 14, 1846–1851 CAS;
(h) V. E. Pashinnik, A. I. Guzyr, A. V. Borovikov and Y. G. Shermolovich, Heteroat. Chem., 2005, 16, 352–356 CrossRef CAS;
(i) E. S. Levchenko, G. S. Borovikova and E. M. Dorokhova, Zh. Org. Khim., 1977, 13, 103–107 CAS.
- B. E. Maryanoff, M. J. Costanzo, S. O. Nortey, M. N. Greco, R. P. Shank, J. J. Schupsky, M. P. Ortegon and J. L. Vaught, J. Med. Chem., 1998, 41, 1315–1343 CrossRef CAS PubMed.
- Both a polar and a radical mechanism have been proposed for the formation imidothionyl chlorides:
(a) I. V. Koval', Russ. J. Org. Chem., 2001, 37, 297–317 CrossRef;
(b) G. N. Dolenko, A. V. Zibarev, S. A. Krupoder, L. N. Mazalov, O. C. Poleshchuk, G. G. Furin, G. F. Khudorozhko and G. G. Yakobson, J. Fluorine Chem., 1981, 17, 1–14 CrossRef CAS.
-
(a) S. Tsuzuki and T. Kano, Angew. Chem., Int. Ed., 2023, 62, e202300637 CrossRef CAS PubMed;
(b) X. Lu, G. Huang, J. Ye, M. A. Bashir, J. Su, K. Yang, F. Liang and X. Xu, Org. Lett., 2023, 25, 2151–2156 CrossRef CAS PubMed;
(c) M. Andresini, M. Spennacchio, M. Colella, G. Losito, A. Aramini, L. Degennaro and R. Luisi, Org. Lett., 2021, 23, 6850–6854 CrossRef CAS PubMed;
(d) F. Boberg, B. Bruchmann, A. Garming and G. Nink, Phosphorus, Sulfur, Silicon Relat. Elem., 1990, 53, 313–326 CrossRef CAS;
(e) A. J. Ruminy and G. Kresze, Phosphorus, Sulfur, Silicon Relat. Elem., 1987, 29, 49–56 CrossRef CAS.
-
(a) A. Ferry, T. Billard, B. R. Langlois and E. Bacqué, J. Org. Chem., 2008, 73, 9362–9365 CrossRef CAS PubMed;
(b) G. Celentano, S. Colonna, N. Gaggero and C. Richelmi, Chem. Commun., 1998, 701–702 RSC;
(c) I. V. Koval', T. G. Oleinik and M. M. Kremlev, Zh. Org. Khim., 1979, 15, 2319–2323 Search PubMed.
- M. Andresini, M. Colella, L. Degennaro and R. Luisi, Org. Biomol. Chem., 2023, 21, 7681–7690 RSC.
- H. S. Veale, J. Levin and D. Swern, Tetrahedron Lett., 1978, 19, 503–506 CrossRef.
- Other S(IV) → S(VI) oxidation methods were screened, involving mCPBA, oxone, KMnO4, SelectFluor, peroxysulfonate, OsO4 or MeReO3 as the oxidizing species, but proved ineffective.
-
(a) R. A. De Marco, T. A. Kovacina and W. B. Fox, J. Fluorine Chem., 1975, 5, 221–230 CrossRef CAS;
(b) T. A. Kovacina, R. A. De Marco, W. B. Fox, D. E. Young and L. R. Anderson, Inorg. Nucl. Chem. Lett., 1974, 10, 763–766 CrossRef CAS.
-
As an example of hydrolytic and thermal stability, Fox reports that bis(trifluoroethyl) sulfite remained completely intact when subjected to water at 125 °C for 16 h, in contrast to dialkyl sulfites which decompose fully at lower temperatures
.
- Other failed nucleophiles include phenol, HOBt, morpholine, and aniline, which may be due to either product stability or the destruction of TsN = SCl2 into unknown compounds (see ESI Section 2.13†).
- The characterization data match very well with the expected spectral data of the proposed sulfamate products. While no alternative chemical synthesis towards these structures was successful, we additionally performed DFT-level predictions of the 1H and 13C NMR chemical shifts, and the high similarity between predicted and experimental NMR spectra further corroborated the identity of the sulfamate product (see ESI Section 2.12†).
- T. J. Maricich, R. A. Jourdenais and T. A. Albright, J. Am. Chem. Soc., 1973, 95, 5831–5832 CrossRef CAS.
-
(a) P. A. Bristow, J. G. Tillett and D. E. Wiggins, J. Chem. Soc. B, 1968, 1360–1363 RSC;
(b) R. E. Davis, J. Am. Chem. Soc., 1962, 84, 599–604 CrossRef CAS.
-
D. R. Klein, Organic Chemistry, Wiley, Hoboken, NJ, 4th edn, 2021 Search PubMed.
- E. S. Levchenko, L. V. Budnik and T. N. Dubinina, Zh. Org. Khim., 1978, 14, 1846–1851 CAS.
- J. L. García Ruano, A. Parra, L. Marzo, F. Yuste and V. M. Mastranzo, Tetrahedron, 2011, 67, 2905–2910 CrossRef.
- J. L. García Ruano, J. Alemán, M. Belén Cid and A. Parra, Org. Lett., 2005, 7, 179–182 CrossRef PubMed.
- Z.-X. Zhang, C. Bell, M. Ding and M. C. Willis, J. Am. Chem. Soc., 2022, 144, 11851–11858 CrossRef CAS PubMed.
|
This journal is © The Royal Society of Chemistry 2024 |
Click here to see how this site uses Cookies. View our privacy policy here.