DOI:
10.1039/D4SC01633E
(Edge Article)
Chem. Sci., 2024,
15, 7603-7609
Norcorroles as antiaromatic π-electronic systems that form dimension-controlled assemblies†
Received
9th March 2024
, Accepted 15th April 2024
First published on 16th April 2024
Abstract
Norcorrole derivatives with 3,4,5-trialkoxyphenyl moieties at the meso positions were synthesized to form various stacking assemblies in single crystals and thermotropic liquid crystals (LCs) depending on aliphatic chain lengths. Triple-decker stacking structures were formed via the interactions between the antiaromatic systems formed for the butoxy and dodecyloxy derivatives in the single-crystal and LC states, respectively. In particular, the LC state exhibited discotic columnar structures comprising triple deckers to exhibit high electric conductivity, as supported by molecular dynamics simulations.
Introduction
The electronic and electrooptical properties emerging from assemblies of π-electronic molecules are controlled by the arrangement of the building units.1 π-Electronic systems form stacking structures via π–π interactions, which are mainly derived from dispersion forces.2 The additional interactions that occur due to peripheral substituents, such as the van der Waals interactions among aliphatic chains, are effective in modulating the stacking structures and resulting properties for applications in molecular electronics.3 In particular, antiaromatic systems with peripheral aliphatic chains are potentially promising candidates for electric conducting materials due to the excellent redox properties derived from the small HOMO–LUMO gaps. However, only a few antiaromatic systems have been considered as the π-electronic building units of dimension-controlled assemblies with stacking structures such as liquid crystals.4 Moreover, their assembling behaviours were similar to those of ordinary aromatic systems because of the small antiaromaticity of the building units.
Norcorroles, porphyrin analogues whose two meso carbons are missing (Fig. 1 top), are promising antiaromatic π-electronic systems for forming stacking structures.5,6 The antiaromatic property of norcorroles is derived from the 16 π-electrons in the conjugated circuit, producing attractive interactions between the antiaromatic systems through stacking, inducing stacked-ring aromaticity, for which face-to-face stacking is crucial.6b,d,g,j A triple-decker structure, stabilized by the stacked-ring aromaticity, was formed in the crystal structure of meso-phenyl-substituted NiII norcorrole, which contained outer units with concave geometries (Fig. 1 right).6b The assembling behaviour was modulated by the introduction of amide-substituted side chains, resulting in the formation of 1D supramolecular polymers with higher charge-carrier mobility than the aromatic counterpart.6h The norcorrole exhibited no obvious near infrared absorption characteristic of the face-to-face stacking in the supramolecular polymer, suggesting the deviation from face-to-face stacked arrangement. Therefore, the norcorrole assemblies through face-to-face stacking in dimension-controlled assemblies have not been achieved to date. The deviation from face-to-face stacked arrangement would be derived from significant hydrogen-bonding interactions between the amide units of side chains. Introduction of other side interacting moieties that have less directionality would enhance the stacking between norcorrole units. This report shows the assembly of norcorroles via the simple introduction of aliphatic chains, which induce van der Waals interactions, to the meso-aryl moieties (Fig. 1 bottom). The synergetic effects of the stacked norcorroles and introduced aliphatic chains were observed in a liquid crystal columnar assembly,7 which exhibited nonconventional nanoscale phase-segregation as a norcorrole-based superstructure, along with enhanced electric conductivity.
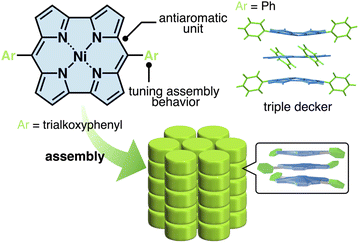 |
| Fig. 1 Dimension-controlled assemblies of NiII norcorroles based on the interactions between antiaromatic systems. | |
Results and discussion
Synthesis and characterization
Norcorrole NiII complexes 1a–e, bearing alkoxy units with different chain lengths at the meso-aryl units, were synthesized from the corresponding dipyrromethanes according to procedures found in the literature (Fig. 2 and S1†).6b NiII, as a diamagnetic d8 state, was selected as a metal centre because it allowed the facile production of norcorrole frameworks. The synthesized compounds were characterized using 1H and 13C NMR and MALDI/ESI-TOF-MS. The 1H NMR of 1a–e in CDCl3 showed antiaromatic properties as suggested by the significant upfield shifts of the β-CH signals (1.95–2.52 ppm) in contrast to those of aromatic porphyrins (9.04–9.18 ppm) (Fig. S9–13 and 17–19†). Their UV/vis absorption spectra in CH2Cl2 exhibited absorption bands at 427, 497 and 524 nm for 1a and 426 and 528 nm for 1b–e, with the alkoxy chains of four or more CH2 units (Fig. S20†). Norcorroles 1a–e exhibited a weak absorption band at up to ∼900 nm, which was characteristic of antiaromatic compounds.6 TD-DFT calculation for the optimized structure of 1a at the B3LYP/6-31G(d,p)-SDD level suggested that the absorption at 519 nm could be assigned to a CT transition band from trialkoxyphenyl units to the norcorrole core part (Fig. S42†).8
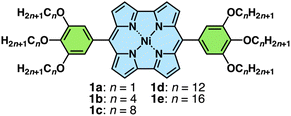 |
| Fig. 2 NiIImeso-(3,4,5-trialkoxyphenyl)norcorroles 1a–e. | |
Upon cooling, the β-CH 1H NMR signals of 1d at 2.51 and 2.07 ppm at 20 °C in CD2Cl2 (1 mM) were shifted downfield to 3.22 and 2.80 ppm, respectively, at −30 °C, suggesting that the stacked-ring aromaticity was induced by stacking of the antiaromatic core (Fig. S77†).6b This result was consistent with the larger values in harmonic oscillator model of aromaticity (HOMA: 0.53, estimated based on the internal ring comprising 14 atoms) and more negative nucleus-independent chemical shift (NICS) estimated for an optimized structure of the double decker of 1a compared to those of the monomeric 1a (HOMA: 0.36) (Fig. S38 and 46†). The diatropic ring current indicated with clockwise arrows in the anisotropy of the induced current density (ACID) plot of the stacked 1a dimer also supported the stacked-ring aromaticity (Fig. S48†).8–11 The UV/vis absorption band of 1d at ∼850 nm, which is characteristic of closely stacked norcorroles,6b,d,g,i,j in CH2Cl2 (1 mM) appeared upon cooling to −40 °C, with drastic changes at short wavelengths, suggesting the aggregation (Fig. S74†). The absorption band in the long wavelength region theoretically supported the formation of an oligomeric aggregate (Fig. S43 and 44†).8 In contrast, 1e rapidly formed precipitates at ∼0 °C. The cyclic voltammogram (CV) of 1b in CH2Cl2 exhibited three reversible oxidation (0.16, 0.40 and 1.07 V vs. Ag/Ag+) and two reversible reduction waves (−0.64 and −1.32 V) in the −2.0 to +1.3 V region (Fig. S21†). The intensities of the first two oxidation waves were approximately half of those of the other redox waves, which was similar to the previously reported results for ortho-unsubstituted meso-arylnorcorroles, suggesting the formation of a mixed-valence dimer in the first oxidation process.6g
Solid-state assemblies
Single crystals of 1a, b were prepared from C6H5Cl/MeOH and used for X-ray analysis.12 In the solid state, 1a had a square planar NiII coordination geometry with the N–Ni distances of 1.78–1.79 Å and a deviation of 0.05 Å for the mean plane (core 23 atoms), to which the meso-aryl rings were tilted at 45.3° (Fig. 3a(i)). In the packing diagram, stacking along the b axis was observed with an interplane distance of 3.67 Å and the meso-aryl units also showed π–π-stacking with an interplane distance of 3.56 Å. The Ni⋯Ni distance and angle of the 1a plane in the columnar direction were 3.93 Å and 69.0°, respectively, with a slip distance of 1.41 Å. The long axis of the 1a crystal corresponded to the b axis in the unit cell, along which the norcorroles were stacked (Fig. 3a(ii) and S29†). On the other hand, X-ray analysis of 1b showed a triple-decker stacking structure (Fig. 3b(i)) similar to that of meso-phenyl-substituted norcorrole.6b In 1b, the centre norcorrole was relatively planar with a deviation of 0.05 Å for the mean plane and staggered by 72.6° relative to the outer stacked molecules. The outer norcorrole units adopted a bowl-shaped conformation and a mean-plane deviation of 0.16 Å. The bowl depth defined by a Ni···mean-plane (eight β-C atoms) distance was 0.49 Å. The Ni⋯Ni distance and that between the two mean planes (four N atoms) of the centre and outer norcorroles were 3.00 and 3.13 Å, respectively. The close stacking of 1b was observed with a larger overlap between the core π-planes compared to 1a. The meso-aryl rings of the centre and outer 1b were tilted at 41.9° and 48.9°/52.4°, respectively, to the mean planes (23 atoms). In the crystal of 1b, the long axis corresponded to the a axis, which was the direction of the columnar assemblies of 1b3 with a tilt (Fig. 3b(ii) and S31†). The HOMA values of 1b (top and centre of triple decker: 0.46 and 0.53, respectively) were larger than that of 1a (0.35), indicating that the triple decker of 1b exhibited the stacked-ring aromaticity in contrast to slip-stacked 1a (Fig. S32†). NICS9,11 values in the norcorrole macrocycles of the crystal structure of 1a (slip-stacked trimer 1a3) at BHLYP/6-31G(d)-SDD level8 were comparable to that of monomeric 1a, whereas that of the crystal structure of 1b (triple decker 1b3, whose butyl groups were replaced with methyl groups) were significantly lower than those of monomeric 1a (Fig. 4a, b, S46 and 47†). In addition, arrows indicating the current density in the ACID plot of 1a3 were directed counterclockwise similar to monomeric 1a, while those on the central norcorrole unit of 1b3 were partially directed clockwise (Fig. 4b and S48†).10 These results supported the contribution of stacked ring aromaticity of 1b3.
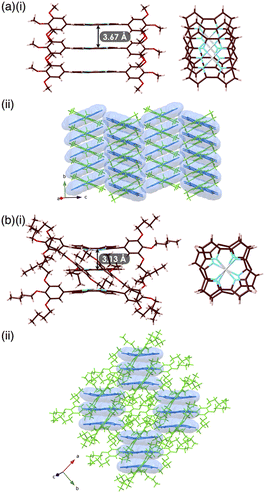 |
| Fig. 3 (a) Single-crystal X-ray structures of (a) 1a and (b) 1b as (i) slip-stacked trimer and triple decker, respectively, showing the distances between two mean planes (side and core top views) and (ii) representative packing structures (blue: core unit, green: aryl unit). Atom colour codes in (i): brown, pink, cyan, red and grey refer to carbon, hydrogen, nitrogen, oxygen and nickel, respectively. | |
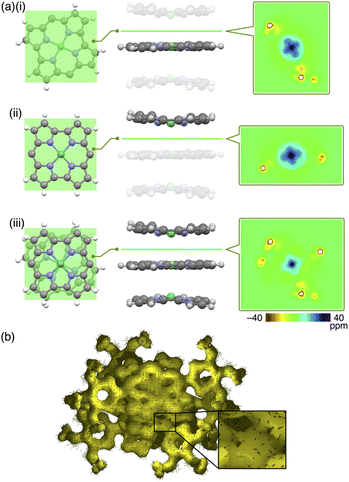 |
| Fig. 4 (a) Arrangements of the norcorrole units in the crystal structure of 1b as top (left) and side (centre) views and 2D NICS plots (right, ppm), estimated for the planes (left) and lines (centre), as (i) monomer (centre), (ii) monomer (top) and (iii) stacked trimer at BHLYP/6-31G(d)-SDD level and (b) ACID plot of 1b trimer in the crystal structure. The translucent parts in the side views in (a) (i, ii) were not included in the calculations. Meso-aryl groups in (a) were omitted for clarity, and the butoxy groups were replaced with methoxy groups for facile calculations. | |
Columnar liquid crystals based on triple deckers
Introduction of aliphatic chains to norcorrole provided mesophases (Fig. 5). Mosaic textures of polarized optical microscopy (POM) were observed for 1d upon cooling from isotropic liquid state (Fig. 5a(i) and S51†). The mesophases formed in the dodecyloxy-substituted 1d had transition temperatures at 10/25/45/69/79 °C (heating) and 48/20/15/–15 °C (cooling) as revealed by differential scanning calorimetry (DSC) and synchrotron X-ray diffraction (XRD), which further revealed the assembled structures in the mesophases (Fig. 6, S49, 57–59 and Table S4†). A schematic diagram of the phase transition behaviour is shown in Fig. 5b(i). In particular, the diffraction peaks were intensified upon heating, suggesting the formation of a highly ordered columnar phase (Fig. S57p–u†). The mesophase at 30 °C upon heating showed a diffraction pattern ascribable to the hexagonal columnar (Colh) phase with the parameters a = 3.15 nm, c = 0.93 nm and Z = 3 (ρ = 0.99) (Fig. 6a(i)), and that at 50 °C upon heating was the rectangular columnar (Colr) phase with the parameters a = 5.62 nm, b = 3.10 nm, c = 0.93 nm and Z = 6 (ρ = 0.99) (Fig. 6a(ii)), which were observed in two separated temperature ranges. The assignment of 0.93 nm as the stacking distance was confirmed by the increased intensity of the diffraction in the shearing direction, in contrast to the augmentation of other diffractions derived from the rectangular pattern in the horizontal direction (Fig. 6b). The value of 0.93 nm, which was included in both phases, could be ascribed to the repeating distance of the stacking trimeric units, as also seen in the crystal structure of 1b (Fig. 3b). In addition, the film-state 1d in the Colr phase at the higher temperature exhibited an absorption band at ∼1130 nm (Fig. S75a†), which was red-shifted compared to those of previously reported stacked dimers and aggregates (λmax = 800–900 nm).6b,d,g,i,j The significantly red-shifted absorption for the 1d film compared to the norcorrole double deckers was consistent with the red-shifted absorption suggested by TD-DFT calculation (Fig. S43 and 44†), supporting the formation of the stacked trimer. In the higher temperature region at 69–79 °C upon heating, high fluidity was observed in the phase, which showed complicated diffractions (Fig. S57w†).
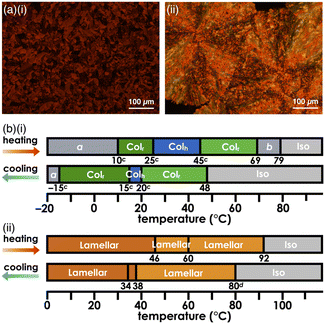 |
| Fig. 5 (a) POM textures of (i) 1d at 40 °C and (ii) 1e at 80 °C upon cooling and (b) schematic diagrams of the phase transition behaviours of (i) 1d and (ii) 1e. Phase a showed no obvious peaks ascribable to the less ordered structure, whereas phase b exhibited a complicated diffraction pattern derived from the unidentified structure. Transition temperatures were determined by DSC without labels and also by XRD and POM labelled with c and d, respectively. Scan rates in DSC were 5 °C min−1 except for 1d at 2 °C min−1, which provided the transition temperatures closer to those determined by XRD. | |
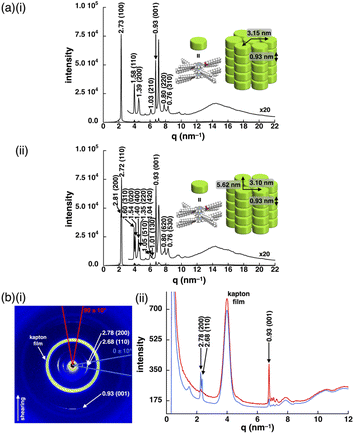 |
| Fig. 6 (a) XRD patterns of 1d at (i) 30 °C and (ii) 50 °C upon heating and possible assembled models and (b) XRD patterns of 1d sheared between Kapton (polyimide) films at approximately 85 °C and cooled to r.t., including (i) 2D XRD diffraction pattern and (ii) a combined diagram showing meridional (sheared) (red) and equatorial (blue) directions. An unassigned peak close to 0.93 nm may be derived from the side aryl moieties. | |
The packing structures of 1d in the Colh and Colr phases at 30 and 50 °C, respectively, were clearly demonstrated in all-atom molecular dynamics (MD) simulations after 100 ns of the equilibration run (Fig. 7). The structures obtained by MD simulation were consistent with those determined by XRD analysis. The concave face of outer norcorrole units in 1d3 were unsuitable for stacking with another triple decker.13 Thus, the triple deckers were not arranged in a straight line in the columnar structures (Fig. 7a right). The assembly mode was distinct from that for conventional discotic columnar structures, where π-electronic parts and aliphatic chains contribute to a 1D ordered assembly, producing more efficient intermolecular interactions and high entropy effects that exhibit fluidity, respectively. In 1d, the proximal locations of the norcorrole units and dodecyl chains were required to convert from the Colh to Colr phase (Fig. 7a and b (left)). In this study, the concave geometries of the norcorrole triple decker resulted in nonconventional assembled structures in combination with aliphatic chains.
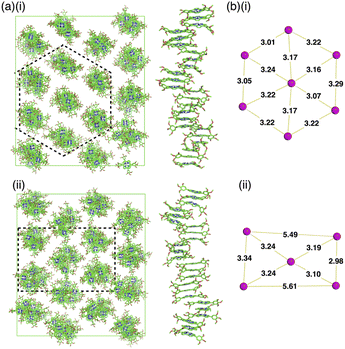 |
| Fig. 7 (a) Snapshots of MD simulation results for 1d and (b) arrangement of the columns with the distances (nm) (the locations are indicated with dashed lines in (a)) after 100 ns of the equilibration run (i) at 30 °C and (ii) 50 °C. The alkyl chains and hydrogens are omitted for clarity. | |
Effects of substituents and frameworks on assembled structures
The lengths of the aliphatic chains were essential factors in controlling the assembled structures. Hexadecyloxy-substituted 1e exhibited the formation of mesophases with transition temperatures of 46/60/92 °C (heating) and 85/38/34 °C (cooling), as revealed by DSC and POM (Fig. 5a, b(ii), S49 and 52†). The synchrotron XRD results showed the formation of lamellar phases with repeating distances of 5.32–5.44 nm, which were consistent with the molecular lengths (Fig. S60 and 61†). The diffractions at ∼0.40 nm instead of that at 0.93 nm were ascribed to the stacking distances of 1e, suggesting that 1e did not form triple deckers in the bulk state in contrast to 1d.14 Compared to norcorroles 1d, e with long aliphatic chains, 1c had a higher melting point at 126 °C, at which it was decomposed upon the conversion to an isotropic liquid (Fig. S49†). The synchrotron XRD results for 1c in the state obtained by recrystallization showed the formation of the Colr phase, with a repeating distance of 0.93 nm (Fig. S54†), which was similar to those of 1b and 1d in the single-crystal and mesophase forms, respectively. In 1c with shorter aliphatic chains, the interactions between the core π-electronic systems were predominant in forming triple deckers and their assemblies, resulting in higher crystallinity. In contrast, 1e, with longer aliphatic chains, exhibited mesophases, wherein the stacking distance between the π units was relatively long (0.40 nm) in the lamellar phases. These longer aliphatic chains more significantly influenced on the formation of assemblies, resulting in difficulty with the close stacking of π units because of the relatively smaller interactions between the core π-electronic systems. On the other hand, 1d with aliphatic chains of a moderate length could also form mesophases, wherein the core π-electronic system played an essential role in the aggregate formation, as Colh and Colr structures with close stacking.
The phase transition behaviours of analogous porphyrins 2c–e with the corresponding aryl moieties to those of 1c–e at the 5,15-positions, respectively, were also examined (Fig. S53b†). In contrast to 1d, dodecyloxy 2d formed a lamellar structure instead of columnar phases (Fig. S64†). Hexadecyloxy 2e formed a lamellar structure, with a repeating distance similar to that of 1e, which was maintained during the cooling and heating processes (Fig. S66†). On the other hand, octyloxy-substituted 2c, which had a lower melting point (68 °C) than 1c, showed no ordering behaviour during the cooling process (Fig. S62†), and a crystal-like POM texture was observed during the heating process (Fig. S50†). These observations suggested that, for the derivatives with longer aliphatic substituents, the interactions between the aliphatic groups were more significant than those between the π-planes, inducing a similar tendency to form lamellar structures. For the derivatives with moderately long aliphatic chains, distinct assembled structures were formed according to the interactions between π-planes in the norcorroles and porphyrins.
Electric conductive property
Owing to the close stacking modes in the Colh and Colr phases of 1d with the wide range of continuous mesophases, the electric conductive nature of the phases was examined using flash-photolysis time-resolved microwave conductivity (FP-TRMC) measurements upon charge carrier photo-injection. The liquid crystalline sample of 1d was investigated after heating to the isotropic liquid state and processes for phase stability at each step. The short-range proximity of the NiII norcorrole framework of crystal-state 1b as a reference was confined to the trimer forms in the condensed phase, disrupting the 1D electric conductive pathways. The advantages of the 1D continuous stacking modes in the mesophases of 1d were clearly shown at 25 °C (Fig. 8). The maximum photo-conductivity of 1d (5.2 × 10−8 m2 V−1 s−1) was over five times of that of 1b and greater than those of other reported norcorrole triple deckers, including that of meso-phenyl derivative, in the form of single crystals.6c,f These observations suggested that alkoxy chains contributed to the arrangement of triple deckers, resulting into the enhanced electric conductivity. In addition, the photo-conductivities of 1d and 1b were significantly larger than those of slip-stacked 1e (liquid crystal) and 1a (crystal), respectively, probably due to the ordered arrangement of tightly stacked triple deckers. The conductivity of 1d was also higher than the previously reported value for a norcorrole supramolecular polymer (1 × 10−8 m2 V−1 s−1).6h Upon heating 1d from 25 °C, partial disruption of the conducting pathways was observed with an initial rapid decay until a few microseconds due to the quenching of electrons via residual oxygen molecules in the system (Fig. S80a†).
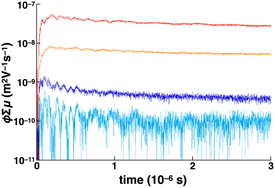 |
| Fig. 8 Kinetic traces of transient photoconductivity observed for 1a (light blue), 1b (orange), 1d (red) and 1e (blue) upon excitation at 355 nm, 2.7 × 1015 photons cm−2, at 20, 20, 25 and 50 °C, respectively. | |
Conclusions
NiII norcorrole derivatives with alkoxy chains having various lengths exhibited unique assembling behaviours and enhanced electric conductivity. The norcorroles with specific alkoxy chains formed triple-decker structures, derived from the stacked-ring aromaticity, in the crystal and mesophase states. As observed in the crystal structure, concave faces of the bowl-shaped top and bottom units of the triple deckers hindered effective stacking of the norcorrole trimer. In addition, the norcorrole trimer core was surrounded by alkyl chains, resulting in a double concave discotic mesogen. In the LC state, the stacked triple-decker structure was in contact with adjacent triple deckers at the edges of both concave faces, providing a novel nanoscale phase-segregated columnar assembly via the interactions between the edges of the norcorrole concave faces, along with those between the core π-electronic units and side aliphatic chains. The assembling modes in the LC states were substantially different from those of ordinary aromatic systems, showing more ordered segregated structures via independent interactions between the core π-electronic units and aliphatic chains. Even a slight overlap between the triple deckers led to high electric conductivity owing to the dynamic behaviours with a suitable arrangement in the LC state. Further modifications of antiaromatic π-electronic systems to obtain functional electronic materials are currently under investigation.
Data availability
Data supporting the work in this publication are available via the ESI† and associated crystallographic data.
Author contributions
H. M. designed and conducted the project. S. I., K. Y., S. Su., Y. H. and Y. B. carried out the synthesis, characterization and property examinations. S. Sa. and G. W. conducted the MD calculations. Y. H. K. and S. Se. evaluated electric conductivities. H. S. supported the synthesis.
Conflicts of interest
There are no conflicts of interest to declare.
Acknowledgements
This work was supported by JSPS KAKENHI Grant Numbers JP18H01968, JP22H02067 and JP23K23335 for Scientific Research (B), JP19K05444 and JP2408389 for Scientific Research (C), JP23K17951 for Challenging Research (Exploratory), JP20H05862, JP20H05863 and JP20H05867 for Transformative Research Areas (A) “Condensed Conjugation”, JP19H05718 for Scientific Research on Innovative Areas “Aquatic Functional Materials”, Ritsumeikan Global Innovation Research Organization (R-GIRO) project (2017–22 and 2022–27), JST SPRING Grant Number JPMJSP2101, JST CREST Grant Number JPMJCR23O1 and the Sasakawa Scientific Research Grant from the Japan Science Society. Theoretical calculations were partially performed using Research Center for Computational Science, Okazaki, Japan (21-IMS-C077, 22-IMS-C043, 22-IMS-C077, 23-IMS-C038 and 23-IMS-C069). We thank Prof. Kunihisa Sugimoto, Kindai University, and Dr Nobuhiro Yasuda, JASRI/SPring-8, for synchrotron-radiation single-crystal X-ray analysis (BL02B1 and BL40XU at SPring-8: 2021A1597 and 2021A1339), Dr Noboru Ohta (JASRI/SPring-8) for synchrotron-radiation XRD measurements (BL40B2 at SPring-8: 2016A1360, 2016B1498, 2020A1586, 2020A1664, 2021A1479, 2021B1828, 2022A1689, 2022B1546, 2023A1331 and 2023B1409), Prof. Takayuki Tanaka and Dr Akito Nakai, Kyoto University, Prof. Osamu Tsutsumi, Ritsumeikan University, for single-crystal X-ray analysis, and Prof. Hitoshi Tamiaki, Ritsumeikan University, for various measurements.
Notes and references
-
(a)
Unimolecular and Supramolecular Electronics I: Chemistry and Physics Meet at Metal–Molecule Interfaces, Topics in Current Chemistry, ed. R. M. Metzger, Springer, 2012 Search PubMed;
(b)
Supramolecular Materials for Opto-Electronics, ed. N. Koch, RSC, 2015 Search PubMed.
- C. A. Hunter and J. K. M. Sanders, J. Am. Chem. Soc., 1990, 112, 5525–5534 CrossRef CAS.
-
(a) S. Laschat, A. Baro, N. Steinke, F. Giesselmann, C. Hägele, G. Scalia, R. Judele, E. Kapatsina, S. Sauer, A. Schreivogel and M. Tosoni, Angew. Chem., Int. Ed., 2007, 46, 4832–4887 CrossRef CAS PubMed;
(b) B. R. Kaafarani, Chem. Mater., 2011, 23, 378–396 CrossRef CAS;
(c) T. Wöhrle, I. Wurzbach, J. Kirres, A. Kostidou, N. Kapernaum, J. Litterscheidt, J. C. Haenle, P. Staffeld, A. Baro, F. Giesselmann and S. Laschat, Chem. Rev., 2016, 116, 1139–1241 CrossRef PubMed;
(d) H. K. Bisoyi and Q. Li, Chem. Rev., 2022, 122, 4887–4926 CrossRef CAS PubMed.
-
(a) S.-i. Kato, N. Takahashi, H. Tanaka, A. Kobayashi, T. Yoshihara, S. Tobita, T. Yamanobe, H. Uehara and Y. Nakamura, Chem.–Eur. J., 2013, 19, 12138–12151 CrossRef CAS PubMed;
(b) N. Takahashi, S. Kato, M. Yamaji, M. Ueno, R. Iwabuchi, Y. Shimizu, M. Nitani, Y. Ie, Y. Aso, T. Yamanobe, H. Uehara and Y. Nakamura, J. Org. Chem., 2017, 82, 8882–8896 CrossRef CAS PubMed.
- M. Bröring, S. Köhler and C. Kleeberg, Angew. Chem., Int. Ed., 2008, 47, 5658–5660 CrossRef PubMed.
-
(a) T. Ito, Y. Hayashi, S. Shimizu, J.-Y. Shin, N. Kobayashi and H. Shinokubo, Angew. Chem., Int. Ed., 2012, 51, 8542–8545 CrossRef CAS PubMed;
(b) R. Nozawa, H. Tanaka, W.-Y. Cha, Y. Hong, I. Hisaki, S. Shimizu, J.-Y. Shin, T. Kowalczyk, S. Irle, D. Kim and H. Shinokubo, Nat. Commun., 2016, 7, 13620 CrossRef CAS PubMed;
(c) T. Yoshida, D. Sakamaki, S. Seki and H. Shinokubo, Chem. Commun., 2017, 53, 1112–1115 RSC;
(d) R. Nozawa, J. Kim, J. Oh, A. Lamping, Y. Wang, S. Shimizu, I. Hisaki, T. Kowalczyk, H. Fliegl, D. Kim and H. Shinokubo, Nat. Commun., 2019, 10, 3576 CrossRef PubMed;
(e) S. Liu, H. Tanaka, R. Nozawa, N. Fukui and H. Shinokubo, Chem.–Eur. J., 2019, 25, 7618–7622 CrossRef CAS PubMed;
(f) S. Ukai, Y. H. Koo, N. Fukui, S. Seki and H. Shinokubo, Dalton Trans., 2020, 49, 14383–14387 RSC;
(g) H. Kawashima, S. Ukai, R. Nozawa, N. Fukui, G. Fitzsimmons, T. Kowalczyk, H. Fliegl and H. Shinokubo, J. Am. Chem. Soc., 2021, 143, 10676–10685 CrossRef CAS PubMed;
(h) S. Ukai, A. Takamatsu, M. Nobuoka, Y. Tsutsui, N. Fukui, S. Ogi, S. Seki, S. Yamaguchi and H. Shinokubo, Angew. Chem., Int. Ed., 2022, 61, e202114230 CrossRef CAS PubMed;
(i) S.-Y. Liu, N. Kishida, J. Kim, N. Fukui, R. Haruki, Y. Niwa, R. Kumai, D. Kim, M. Yoshizawa and H. Shinokubo, J. Am. Chem. Soc., 2023, 145, 2135–2141 CrossRef CAS PubMed;
(j) S. Kino, S. Ukai, N. Fukui, R. Haruki, R. Kumai, Q. Wang, S. Horike, Q. M. Phung, D. Sundholm and H. Shinokubo, J. Am. Chem. Soc., 2024, 146, 9311–9317 CrossRef CAS PubMed.
- Examples for liquid crystals of porphyrinoids:
(a) P. G. Schouten, J. F. Van Der Pol, J. W. Zwikker, W. Drenth and S. J. Picken, Mol. Cryst. Liq. Cryst., 1991, 195, 291–305 CrossRef CAS;
(b) M. Ichihara, A. Suzuki, K. Hatsusaka and K. Ohta, Liq. Cryst., 2007, 34, 555–567 CrossRef CAS;
(c) M. Stępień, B. Donnio and J. L. Sessler, Chem.–Eur. J., 2007, 13, 6853–6863 CrossRef PubMed;
(d) D. Gao, J. A. Edzang, A. K. Diallo, T. Dutronc, T. S. Balaban, C. Videlot-Ackermann, E. Terazzi and G. Canard, New J. Chem., 2015, 39, 7140–7146 RSC.
-
M. J. Frisch, et al.Gaussian 16, Revision C.01, Gaussian, Inc., Wallingford CT, 2016 Search PubMed.
- P. v. R. Schleyer, C. Maerker, A. Dransfeld, H. Jiao and N. J. R. van Eikema Hommes, J. Am. Chem. Soc., 1996, 118, 6317–6318 CrossRef CAS PubMed.
-
(a) R. Herges and D. Geuenich, J. Phys. Chem. A, 2001, 105, 3214–3220 CrossRef CAS;
(b) D. Geuenich, K. Hess, F. Köhler and R. Herges, Chem. Rev., 2005, 105, 3758–3772 CrossRef CAS PubMed.
- T. Lu and F. Chen, J. Compt. Chem., 2012, 33, 580–592 CrossRef CAS PubMed.
- For the synchrotron radiation measurements:
(a) N. Yasuda, H. Murayama, Y. Fukuyama, J. E. Kim, S. Kimura, K. Toriumi, Y. Tanaka, Y. Moritomo, Y. Kuroiwa, K. Kato, H. Tanaka and M. Takata, J. Synchrotron Radiat., 2009, 16, 352–357 CrossRef CAS PubMed;
(b) N. Yasuda, Y. Fukuyama, K. Toriumi, S. Kimura and M. Takata, AIP Conf. Proc., 2010, 1234, 147–150 CrossRef CAS;
(c) K. Sugimoto, H. Ohsumi, S. Aoyagi, E. Nishibori, C. Moriyoshi, Y. Kuroiwa, H. Sawa and M. Takata, AIP Conf. Proc., 2010, 1234, 887–890 CrossRef CAS.
- Mesophases of triple-decker complexes comprising porphyrins and phthalocyanines:
(a) H. Miwa, N. Kobayashi, K. Ban and K. Ohta, Bull. Chem. Soc. Jpn., 1999, 72, 2719–2728 CrossRef CAS;
(b) T. Nakai, K. Ban, K. Ohta and M. Kimura, J. Mater. Chem., 2002, 12, 844–850 RSC;
(c) Y. Zhang, W. Jiang, J. Jiang and Q. Xue, J. Porphyrins Phthalocyanines, 2007, 11, 100–108 CrossRef CAS;
(d) H. Mukai, K. Hatsusaka and K. Ohta, J. Porphyrins Phthalocyanines, 2007, 11, 846–856 CrossRef CAS;
(e) Y. Zhang, J. Jiang, X. Sun and Q. Xue, Aust. J. Chem., 2009, 62, 455–463 CrossRef CAS.
- In contrast to 1d, 1e in the film state exhibited an absorption band at 849 nm without an obvious band at ∼1130 nm, suggesting the formation of the stacked dimer which was not ordered in each layer in the lamellar phases.
Footnote |
† Electronic supplementary information (ESI) available: Synthetic procedures, analytical data, computational details and CIF files for the single-crystal X-ray structural analysis. CCDC 2288764–2288767. For ESI and crystallographic data in CIF or other electronic format see DOI: https://doi.org/10.1039/d4sc01633e |
|
This journal is © The Royal Society of Chemistry 2024 |
Click here to see how this site uses Cookies. View our privacy policy here.