DOI:
10.1039/D4AY01922A
(Paper)
Anal. Methods, 2025,
17, 178-183
A rhodamine based near-infrared fluorescent probe for selective detection of Cu2+ ions and its applications in bioimaging†
Received
21st October 2024
, Accepted 12th November 2024
First published on 13th November 2024
1. Introduction
Copper is the third most abundant transition metal in the human body. An imbalance of copper is associated with several severe diseases, including Alzheimer's disease, prion diseases, amyotrophic lateral sclerosis, neurodegenerative diseases, and cancer.1 Under physiological conditions, copper maintains stable oxidation and reduction states. Monovalent copper ions preferentially bind to softer thioether-rich ligands, whereas divalent copper ions are inclined to associate with harder ligands containing nitrogen and oxygen atoms. Leveraging these properties, a range of fluorescent probes have been engineered.2–10
Rhodamine dyes possess exemplary photophysical properties, which include a high molar extinction coefficient, a substantial quantum yield, and resilience to photobleaching. A notable characteristic of rhodamine dyes is the tunability of their fluorescence through reversible ring-opening/closing mechanisms or Photoinduced Electron Transfer (PET) effects.11–13 However, the absorbance and emission wavelengths of classical rhodamine-based fluorescent probes, such as rhodamine B, rhodamine 6G, and rhodamine 101, are confined to the visible spectrum (500–600 nm). This range limits their applicability for in vivo biological imaging. To address this limitation, researchers have synthesized near-infrared rhodamine analogs through chemical modification of the xanthene core.14–23
Given the limitations of rhodamine, we synthesized a near-infrared fluorescence probe, TM2 (see Scheme 1), which exhibits specific recognition of Cu2+ by integrating a quinoline analog (known for its strong affinity for metal ions) with the rhodamine scaffold. TM2 has proven effective in detecting Cu2+ concentrations in both cellular and murine environments.
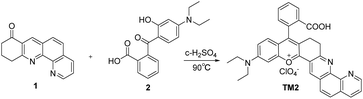 |
| Scheme 1 Synthesis of probe TM2. | |
2. Experimental
2.1. Materials and instruments
Unless otherwise stated, all chemical reagents were of analytical grade, commercially available and used without further purification. The stock solutions of ions were prepared from FeCl2, MgCl2, NiCl2, PbCl2, AlCl3, CrCl3, AgCl, BaCl2, CoCl2, MnCl2, HgCl2, ZnCl2, LiCl, NaCl, KCl, CaCl2, FeCl3·6H2O, CuCl, CuCl2, CdCl2·H2O, H2S, H2O2, NaHCO3, and NaHS with doubly distilled water. 1H NMR (500 MHz) and 13C NMR (125 MHz) were measured on a Bruker Avance III spectrometer. Electrospray mass spectra (ESI-MS) were recorded on a Thermo Fisher LCQ. High-resolution mass spectra were recorded on a HPLC-Q-TOF MS (Micro) spectrometer. Fluorescence spectra were determined on a PerkinElmer LS-55. UV-vis spectra were measured on a Shimadzu UV-3600. Confocal fluorescence imaging studies were performed on a Leica SP8 STED 3× laser confocal fluorescence microscope. The excitation imaging for labile copper fluctuation in mice was realized using an IVIS Lumina K Series III instrument (PerkinElmer). The pH values of sample solutions were monitored using a PHS-3 system. TLC analysis was performed on silica gel plates and column chromatography was conducted over silica gel (mesh 200–300), both of which were obtained from the Qingdao Ocean Chemicals. The data of absolute fluorescence quantum yield and fluorescence lifetime were obtained using a Horiba Fluorolog-QM steady-state and time-resolved fluorescence phosphorescence spectrometer from Canada.
2.2. Synthesis of probe TM2
The synthetic route towards TM2 is depicted in Scheme S1† and characterization of new compounds is described in the ESI (Fig. S6–S10).† Compounds 2 and 1 were prepared according to previous reports with modifications,24–27 and detailed synthesis procedures of the compounds 1, 2, and TM2 are described in the ESI.†
2.3. General procedure for UV-vis and fluorescence studies
Stock solutions of metal ions were prepared (1.0 × 10−2 mol L−1) in phosphate buffer solution (pH = 6.8). A stock solution of compound TM2 (1.0 × 10−4 mol L−1) was prepared in ethanol and then diluted to 10 μM with ethanol/H2O (5
:
5, v/v) immediately before the experiments. In titration experiments, a 2 mL solution of compound TM2 (10 μM) was filled in a quartz cuvette of 1 cm optical path length, and then the Cu2+ stock solution was added into the quartz cuvette by using a micro-pipette. For fluorescence measurements, excitation was performed at 550 nm, and emission was collected from 560 nm to 900 nm. The slit width was 2 nm for both excitation and emission.
2.4. Cell culture and confocal imaging
HeLa cells were purchased from the American Type Culture Collection. Cells were cultured in a humidified 37 °C, 5% CO2 incubator and maintained in DMEM medium supplemented with 10% fetal bovine serum (FBS), 0.1 mg per mL streptomycin and 100 U per mL penicillin. Confocal fluorescence imaging studies were performed on a Leica SP8 STED 3× laser confocal fluorescence microscope. The working solution was prepared from a 10 mM DMSO solution of compound TM2 and then it was diluted with PBS to a final concentration of 10 μM. Prior to imaging, the medium was removed and cell imaging was carried out after washing cells with PBS three times.
2.5.
In vivo optical imaging of mice
BALB/c mice (8 week-old, female) of specific pathogen free (SPF) grade were purchased from GemPharmatech Co., Ltd., license no. SCXK (Jiangsu) 2018-0008. The mice were housed in animal-holding units in a pathogen-free environment at 22 ± 2 °C and 55 ± 5% humidity. The animal experiments in this study were approved by the Institutional Animal Care and Use Committees of GemPharmatech, approval no. SYXK (Jiangsu) 2018-0027. Mice were anesthetized prior to injection and during imaging via inhalation of isoflurane. The excitation imaging for labile copper fluctuation in mice was realized using an IVIS Lumina K Series III instrument (PerkinElmer). Other details are given in the related figure captions.
3. Results and discussion
3.1. Synthesis and characterization of compound TM2
The target rhodamine-like compound, TM2 (as shown in Scheme 1), was synthesized with a high yield (90%) from straightforward starting materials 1 and 2. The successful synthesis of TM2 was confirmed by 1H NMR, 13C NMR, and mass spectrometry analyses (ESI†).
3.2. Spectral performance
The UV-vis absorption spectrum of probe TM2 was investigated first. As shown in Fig. S1(a),† a pronounced maximum UV-vis absorption peak at 550 nm was observed. Upon excitation at this wavelength, TM2 exhibits a fluorescent emission with a peak at 650 nm (Fig. S1(b)†). This emission peak is redshifted by 50 nm relative to pure rhodamine, showcasing a substantial Stokes shift of 100 nm.
To ascertain the optimal solvent ratio for further assays, we investigated the UV absorption and fluorescence spectra of probe TM2 across varying ethanol/water mixtures. As shown in Fig. S1(c),† when the water proportion was augmented, the absorbance at the characteristic peak of 550 nm progressively increased, culminating at an ethanol/water ratio of 4
:
6. This enhancement can be attributed to the amphiphilic nature of TM2, where its overall hydrophilicity allows for better solubility in increased water content, thereby elevating the absorbance. During these evaluations, the concentration of TM2 was consistently maintained at 10 μM with a fixed excitation of 550 nm. In a purely ethanol environment, the probe exhibited a fluorescence emission peak at 610 nm. However, as the water content was progressively raised from 10% to 100%, there was a noticeable red shift in the emission peak accompanied by a diminishing intensity, as depicted in Fig. S1(d).† Taking into consideration both the proximity of the emission wavelength to the near-infrared region and the satisfactory emission intensity, an ethanol/water ratio of 5
:
5 (v/v) was selected for subsequent experimentation. The steady-state quantum yield and the ideal lifetime were determined by absolute fluorescence quantum yield measurements, specifically using a Horiba Fluorolog-QM fluorescence phosphorescence spectrometer.28,29 The absolute fluorescence quantum yield of the probe TM2 was found to be 0.25, with a lifetime of 1.5 ns, as illustrated in Fig. S11,† in an ethanol/water mixture (5
:
5, v/v).
Subsequent to this, fluorescence titration experiments were performed, as depicted in Fig. 1(a). Upon excitation of the probe at 550 nm, a gradual decrease in fluorescence intensity at 650 nm was observed as the concentration of Cu2+ incrementally increased. The fluorescence intensity plummeted to its nadir with the addition of 5 equivalents of Cu2+, where the attenuation was nearly 100
000 fold. The relationship between the Cu2+ concentration and fluorescence intensity from 2.5 to 8 μM exhibited a strong linear correlation, boasting a coefficient of determination exceeding 99.5%. The detection limit for Cu2+ was established at 2.3 μM, as demonstrated in Fig. 1(b). According to the US Environmental Protection Agency, the maximum permissible concentration of copper ions in drinking water is set at 20 μM. Probe TM2 exhibits exceptional sensitivity in detecting micromolar concentrations of Cu2+ within drinking water and ecological systems. Furthermore, the probe demonstrates a broad detection spectrum for Cu2+, spanning from 0 to 50 μM. Using the external standard method, samples from laboratory tap water and a nearby lake were prepared as solutions with an ethanol/water ratio of 1
:
1. The concentrations of Cu2+ in the tap water and lake water were determined to be 0.7 μmol and 3.0 μmol, respectively, both of which are below the standard permissible limits.
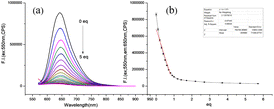 |
| Fig. 1 (a) Fluorescence spectra of compound TM2 (10 μM) towards Cu2+ ions (0–5 equiv.) in ethanol/H2O (5 : 5, v/v) medium. (b) Fluorescence changes of compound TM2 (10 μM) upon addition of Cu2+ (0 to 5 equiv.) (λex: 550 nm). | |
3.3. The selectivity of TM2 to Cu2+
The selectivity and competitive discernment between analytes and various metal ions are crucial metrics for evaluating the efficacy of fluorescent probes. The fluorescence response of the probe TM2 to various cations is illustrated in Fig. 2(a). Prior to the introduction of Cu2+, a TM2 solution (10 μM) excited at 550 nm displayed a fluorescence emission peak at 650 nm. Notably, the addition of Cu2+ alone elicited a substantial alteration in the emission spectrum, whereas the influence of other ions was negligible. Upon the addition of Cu2+ at a concentration 20 times the equivalent, the fluorescence at 650 nm was effectively quenched. This observation underscores the probe's ability to specifically detect Cu2+ and its high selectivity for this ion.
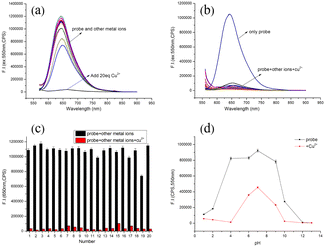 |
| Fig. 2 (a) Fluorescence spectra of compound TM2 (10 μM) with different metal ions (200 μM, 20 equiv.) in ethanol/H2O (5 : 5, v/v) medium for 5 minutes. (b) Fluorescence spectra of compound TM2 (10 μM) with different ions (200 μM, 20 equiv.) and addition of 200 μM of Cu2+ in ethanol/H2O (5 : 5, v/v) medium for 10 minutes. (c) Fluorescence responses of TM2 (10 μM) to various cations in ethanol/H2O (5 : 5, v/v) medium. The black bars represent the TM2 emission intensities in the presence of 200 μM of alkali (IA) and alkaline earth (IIA) metals and for other cations of interest ((1) no ions, (2) H2S, (3) Fe2+, (4) K+, (5) Fe3+, (6) Cd2+, (7) Mg2+, (8) Ni2+, (9) Pb2+, (10) Al3+, (11) NaHS, (12) H2O2, (13) NaHCO3, (14) Cr3+, (15) Ag+, (16) Ba2+, (17) Co2+, (18) Mn2+, (19) Hg2+, (20) Zn2+). The red bars represent the change of the emission that occurs upon the subsequent addition of 200 μM of Cu2+ to the above solution. The intensities were recorded at 650 nm, excitation at 550 nm. (d) Effect of pH on the reaction of compound TM2 (10 μM) with Cu2+ (200 μM, 20 equiv.) in ethanol/H2O (5 : 5, v/v) medium for 10 minutes (λex: 550 nm, λem: 650 nm). | |
The selectivity and resistance to interference from other metal ions are crucial factors in assessing the efficacy of fluorescent probes. The quinoline-like recognition group within the TM2 molecule structure suggests a high affinity for metal ions. Consequently, we examined the impact of coexisting cations on the probe's recognition of Cu2+. The reduction in fluorescence induced by Cu2+ remained unaffected (as depicted in Fig. 2(b)), indicating minimal interference from other metal ions throughout the recognition process. These findings suggest that the probe TM2 exhibits a robust specificity for Cu2+, with limited influence from competing cations. This phenomenon is further illustrated in Fig. 2(c), where the emission intensity at 650 nm exclusively decreases upon the addition of Cu2+, exhibiting negligible impact from other ions. In conclusion, TM2 exhibits exceptional selectivity and anti-interference capabilities for the detection of Cu2+.
3.4. Effect of pH
In addition to the selectivity for metal ions, the pH of the solution is a crucial parameter influencing the recognition performance of the probe. We explored how variations in pH impact the fluorescence response of TM2 to Cu2+. As illustrated in Fig. 2(d), probe TM2 maintained stability and exhibited robust fluorescence intensity across a broad pH spectrum (pH = 5.0–9.0). Within this pH range, the fluorescence response of probe TM2 to Cu2+ remained unaffected by pH fluctuations. This stability suggests that probe TM2 is well-suited for both qualitative and quantitative analysis of Cu2+ concentrations in biological environments.
3.5. Responding mechanism of the probe TM2
To delve deeper into the stoichiometric relationship between TM2 and Cu2+, we graphed the fluorescence intensity of the probe at 650 nm against the ratio [Cu2+]/[TM2 + Cu2+] to produce Fig. S2.† The inflection point materialized at a ratio of [Cu2+]/[TM2 + Cu2+] = 0.5, signifying that probe TM2 engages in a 1
:
1 coordination reaction with Cu2+.30–32
As shown in Fig. S3,† upon the interaction between probe TM2 and Cu2+, the fluorescence emission peak remains unchanged even after the introduction of 80 equivalents of H2S. This constancy suggests either an irreversible recognition process or a substantial binding constant, indicative of a stable complex. To unravel the probe's recognition mechanism, 1H NMR titration experiments were performed, revealing pronounced alterations in the magnetic resonance signals pre- and post-coordination. The discrete non-equivalent nuclear magnetic environments range from 7 to 9 ppm before coordination (Fig. 3). The addition of Cu2+ induced a fusion of the separated signals, attributable to coordination effects. Specifically, the signals at 7.8 ppm, 7.9 ppm, and 8.0 ppm consolidated into a single peak at 8.0 ppm, while the peaks at 8.8 ppm and 9.0 ppm shifted to 8.9 ppm. Furthermore, LC-MS analysis after the addition of 0.5 equivalents of Cu2+ unveiled new chromatographic peaks alongside the TM2 peak. The mass spectral data of this new entity corresponded to TM2, suggesting that the TM2–Cu2+ complex may have been prone to dissociation during ionization,33 as inferred from Fig. S4.†
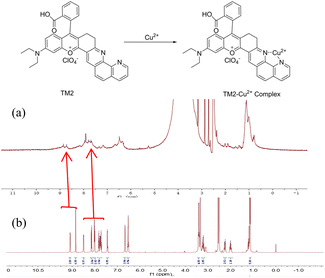 |
| Fig. 3 Proposed sensing mechanism and partial 1H NMR spectra of TM2 (1 mM) in d6-DMSO upon addition of (a) 1 equiv. of Cu2+, (b) 0 equiv. of Cu2+. | |
3.6. Application of probes in water samples
As depicted in Fig. S5,† the introduction of common metal cations, excluding Cu2+, did not elicit discernible color alterations perceptible to the unaided eye. However, upon the addition of Cu2+, the initial purple hue of the TM2 solution under natural light diminished, with a more pronounced color shift observed in the ultraviolet spectrum. This conspicuous color transformation is readily observable by the human eye and holds promise for the in situ detection of Cu2+ in environmental water samples, utilizing merely a portable ultraviolet lamp, thereby circumventing the necessity for intricate instruments and equipment. This approach exhibits potential applicability in the realm of environmental conservation.
3.7. Fluorescence imaging of TM2 in cells
To assess the cytotoxicity of probe TM2, HeLa cells were incubated for 24 hours with varying concentrations of TM2 ranging from 5 to 12 μM. No discernible impact on cell proliferation was noted (Fig. 4(a)). Given the exceptional in vitro characteristics of TM2, its performance in living cells was subsequently investigated. HeLa cells were exposed to TM2 at a concentration of 20 μM for 30 minutes, which resulted in robust intracellular fluorescence. Following the addition of Cu2+ at 20 μM and subsequent incubation for 1–3 hours, a marked reduction in fluorescence was observed under identical conditions (Fig. 4(b)). These pronounced fluorescence alterations suggest that TM2 can effectively penetrate the cell membrane, facilitating the imaging of Cu2+ within living cells.
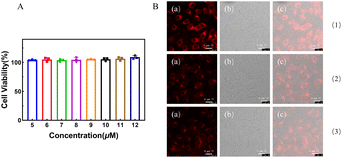 |
| Fig. 4 (A) Cell viability (%) estimated by MTT proliferation tests versus incubation concentrations of TM2. (B) Confocal fluorescence images of living HeLa cells: (1a) cells loaded with 10 μM probe TM2 for 30 min. (1b) Bright field image of (1a). (1c) Overlaid image of panels (1a and 1b). (2a) Probe-loaded cells treated with 20 μM Cu2+ for 1 h. (2b) Bright field image of (2a). (2c) Overlaid image of panels (2a and 2b). (3a) Probe-loaded cells treated with 20 μM Cu2+ for 3 h. (3b) Bright field image of (3a). (3c) Overlaid image of panels (3a and 3b) (λex: 550 nm, λem: 600–750 nm). | |
3.8. Fluorescence imaging of TM2 in mice
Leveraging its favorable near-infrared (NIR) optical properties, TM2 was employed to track Cu2+ in live mice. Experiments utilized BALB/c female mice aged 8 weeks. The mice received subcutaneous injections of TM2 (0.1 mL, 0.3 mg mL−1 in physiological saline) bilaterally, yielding a pronounced fluorescence signal. Following the administration of Cu2+ (0.1 mL, 0.5 mM), a marked decrease in fluorescence intensity was observed. In contrast, the control site injected with PBS buffer exhibited no significant change in fluorescence intensity (Fig. 5). These findings suggest that TM2 could potentially be utilized for monitoring and imaging Cu2+ in living animals.
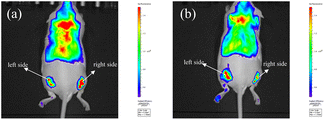 |
| Fig. 5 Fluorescent images of living mice (a) injected with probe TM2 on both sides and (b) preinjected with TM2 on both sides, and then injected with PBS on the left side, injected with Cu2+ on the right side (λex: 560 nm, λem: 620 nm). | |
4. Conclusion
We have successfully synthesized a novel large Stokes shift (100 nm) rhodamine-based near-infrared probe TM2, with the integration of the rhodamine skeleton together with the conjugated bipyridine unit. This probe exhibits excellent selectivity and anti-interference capabilities for Cu2+ recognition in vitro. The Cu2+ detection mechanism is stoichiometric, characterized by high sensitivity and a detection limit of 2.3 × 10−6 mol L−1. The linear detection range is extensive, spanning from 2.5 to 8 μM, with the probe forming a 1
:
1 complex with Cu2+. Furthermore, TM2 can be effectively employed for imaging Cu2+ in HeLa cells and mice.
Data availability
The authors declare that the data supporting the findings of this study are available within the paper and its ESI.† Should any raw data files be needed in another format they are available from the corresponding author upon reasonable request.
Author contributions
Zhijian Chang: synthesis, investigation, results discussion, and writing – original draft. Shumeng Li: investigation, results discussion and writing – reviewing. Fuyan Lin: validation and results discussion. Jia-Hai Ye: supervision, writing – reviewing, editing, and funding acquisition. Yuncong Chen, Zijian Guo, Xuedong Gong: supervision, writing – reviewing.
Conflicts of interest
There are no conflicts to declare.
Acknowledgements
This work was financially supported by the National Natural Science Foundation of China (grant numbers: 21271100 and 91213305) and Priority Academic Program Development of Jiangsu Higher Education Institutions (PAPD).
Notes and references
- G. Sfrazzetto, C. Satriano, G. Tomaselli and E. Rizzarelli, Synthetic fluorescent probes to map metallostasis and intracellular fate of zinc and copper, Coord. Chem. Rev., 2016, 311, 125–167 CrossRef.
- J. Cotruvo, A. Aron, K. Ramos-Torres and C. Chang, Synthetic fluorescent probes for studying copper in biological systems, Chem. Soc. Rev., 2015, 44, 4400–4414 RSC.
- A. Aron, K. Ramos-Torres, J. Cotruvo and C. Chang, Recognition- and Reactivity-Based Fluorescent Probes for Studying Transition Metal Signaling in Living Systems, Acc. Chem. Res., 2015, 48, 2434–2442 CrossRef.
- H. Mbatia and S. Burdette, Photochemical tools for studying metal ion signaling and homeostasis, Biochemistry, 2012, 51, 7212–7224 CrossRef PubMed.
- C. Ackerman, S. Lee and C. Chang, Analytical Methods for Imaging Metals in Biology: From Transition Metal Metabolism to Transition Metal Signaling, Anal. Chem., 2017, 89, 22–41 CrossRef CAS.
- W. Li, W. Qu, X. Zhu, Q. Li, H. Zhang, H. Yao, Q. Lin, Y. Zhang and T. Wei, A highly selective colorimetric and “Off-On” fluorescence sensor for CN- based on Zn(salphenazine) complex, Sci. China: Chem., 2017, 60, 754–760 CrossRef CAS.
- P. Li, X. Duan, Z. Chen, Y. Liu, T. Xie, L. Fang, X. Li, M. Yin and B. Tang, ChemInform Abstract: A Near-Infrared fluorescent probe for detecting copper(II) with high selectivity and sensitivity and its biological imaging applications, Chem. Commun., 2011, 47, 7755–7757 RSC.
- X. Gu and Z. Fang, A novel Hg(2+)-selective fluorescent chemprobe based on thiooxorhodamine-B and β-C-glycoside, Spectrochim. Acta, Part A, 2017, 173, 495–501 CrossRef PubMed.
- S. Goswami, D. Sen, N. Das and G. Hazra, Highly selective colorimetric fluorescence sensor for Cu2+: cation-induced switching on of fluorescence due to excited state internal charge transfer in the red/near-infrared region of emission spectra, Tetrahedron Lett., 2010, 51, 5563–5566 CrossRef.
- H. Zhang, L. Feng, Y. Jiang, Y. Wong, Y. He, G. Zheng, J. He, Y. Tan, H. Sun and D. Ho, A reaction-based near-infrared fluorescent sensor for Cu2+ detection in aqueous buffer and its application in living cells and tissues imaging, Biosens. Bioelectron., 2017, 94, 24–29 CrossRef PubMed.
- Y. Liu, Q. Su, M. Chen, Y. Dong, Y. Shi, W. Feng, Z. Wu and F. Li, Near-Infrared upconversion chemodosimeter for in vivo detection of Cu2+ in wilson disease, Adv. Mater., 2016, 28, 6625–6630 CrossRef PubMed.
- Z. Xu, S. Zheng, J. Yoon and D. Spring, Discovery of a highly selective turn-on fluorescent probe for Ag+, Analyst, 2010, 135, 2554–2559 RSC.
- K. Swamy, H. Kim, J. Soh, Y. Kim, S. Kim and J. Yoon, Manipulation of fluorescent and colorimetric changes of fluorescein derivatives and applications for sensing silver ions, Chem. Commun., 2009, 10, 1234–1236 RSC.
- L. Ma, G. Xu, X. Deng, D. Wen and Y. Yu, A dual-channel chemosensor based on rhodamine and BODIPY conjugated dyad for ratiometric detection of Hg2+ and fluorescence on–off recognition of Cu2+ in aqueous solution and living cells, Chem. Pap., 2023, 77, 583–593 CrossRef.
- W. Lai, Y. Lin, T. Ye, Y. Yu, H. Zhou, L. Li, G. Mao and J. Wang, A Novel Near-Infrared Ratiometric Fluorescence Probe for Hg2+ Based on Quinoline-Fused Rhodamine Dye, J. Fluoresc., 2023, 33, 1413–1419 CrossRef.
- P. Yin, Q. Niu, J. Liu, T. Wei, T. Hu, T. Li, X. Qin and J. Chen, A new AIEE-active carbazole based colorimetric/fluorimetric chemosensor for ultra-rapid and nano-level determination of Hg2+ and Al3+ in food/environmental samples and living cells, Sens. Actuators, B, 2021, 331, 129418 CrossRef.
- D. Aydin, I. Gunay and O. Alici, Highly selective thiazole-appended “switch off” fluorogenic sensor with a detection level of nM for Cu2+: DFT calculations and practically applications, J. Lumin., 2022, 242, 118561 CrossRef.
- Y. Yuan, S. Sun, S. Liu, X. Song and X. Peng, Highly sensitive and selective turn-on fluorescent probes for Cu2+ based on rhodamine B, J. Mater. Chem. B, 2015, 3, 5261–5265 RSC.
- W. Huang, C. Song, C. He, G. Lv, X. Hu, X. Zhu and C. Duan, Recognition Preference of Rhodamine-Thiospirolactams for Mercury(II) in Aqueous Solution, Inorg. Chem., 2009, 48, 5061–5072 CrossRef PubMed.
- J. Liu, Y. Sun, P. Wang, J. Zhang and W. Guo, Construction of NIR and ratiometric fluorescent probe for Hg2+ based on a rhodamine-inspired dye platform, Analyst, 2013, 138, 2654–2660 RSC.
- N. Georgiev, M. Dimitrova, A. Asiri, K. Alamry and V. Bojinov, Synthesis, sensor activity and logic behaviour of a novel bichromophoric system based on rhodamine 6G and 1,8-naphthalimide, Dyes Pigm., 2015, 115, 172–180 CrossRef.
- H. Lv, S. Huang, B. Zhao and J. Miao, A new rhodamine B-based lysosomal pH fluorescent indicator, Anal. Chim. Acta, 2013, 788, 177–182 CrossRef PubMed.
- H. Li, H. Guan, X. Duan, J. Hu, G. Wang and Q. Wang, An acid catalyzed reversible ring-opening/ring-closure reaction involving a cyano-rhodamine spirolactam, Org. Biomol. Chem., 2013, 11, 1805–1809 RSC.
- M. Tamura, H. Ogata, Y. Ishida and Y. Takahashi, Design and synthesis of chiral 1,10-phenanthroline ligand, and application in palladium catalyzed asymmetric 1,4-addition reactions, Tetrahedron Lett., 2017, 58, 3808–3813 CrossRef.
- R. Zibaseresht, P. Karimi, S. Mohit-Azar, M. Amirloo and M. Azimi, An improved synthesis of 2-(pyrid-2’-yl)-1,10-phenanthroline tridentate terpyridyl ligand, Int. J. Chem. Res., 2013, 5, 153–158 CrossRef.
- Y. Hu, Q. Xiang and R. Thummel, Bi-1,10-phenanthrolines and Their Mononuclear Ru(II) Complexes, Inorg. Chem., 2002, 41, 3423 CrossRef.
- H. Wang, J. Guan, X. Han, S. Chen, T. Li, Y. Zhang, M. Yuan and J. Wang, Benzothiazole modified rhodol as chemodosimeter for the detection of sulfur mustard simulant, Talanta, 2018, 189, 39–44 CrossRef.
- Z. Wei, M. Xu, J. Liu, W. Guo, Z. Jiang and W. Shangguan, Simultaneous visible-light-induced hydrogen production enhancement and antibiotic wastewater degradation using MoS2@ZnxCd1-xS: solid-solution-assisted photocatalysis, Chin. J. Catal., 2020, 41, 103–113 CrossRef CAS.
- A. Aisikaer, M. Sa, Y. Jin, Y. Che and X. Li, A novel 1,3-diazepine-based fluorescent probe for highly selective detection of Hg2+, Dyes Pigm., 2024, 227, 112170 CrossRef.
- Y. Yang, W. Gao, R. Sheng, W. Wang, H. Liu, W. Yang, T. Zhang and X. Zhang, Rhodamine-based derivatives for Cu2+ sensing: spectroscopic studies, structure-recognition relationships and its test strips, Spectrochim. Acta, Part A, 2011, 81, 14–20 CrossRef PubMed.
- R. Sheng, P. Wang, W. Liu, X. Wu and S. Wu, A new colorimetric chemosensor for Hg2+ based on coumarin azine derivative, Sens. Actuators, B, 2008, 128, 507–511 CrossRef.
- Y. Zhao, X. Zhang, Z. Han, L. Qiao, C. Li, L. Jian, G. Shen and R. Yu, Highly Sensitive and Selective Colorimetric and Off–On Fluorescent Chemosensor for Cu2+ in Aqueous Solution and Living Cells, Anal. Chem., 2009, 81, 7022–7030 CrossRef PubMed.
- A. Bond, R. Colton, A. D'Agostino, A. Downard and J. Traeger, A Role for Electrospray Mass Spectrometry in Electrochemical Studies, Anal. Chem., 1995, 67, 1691–1695 CrossRef.
|
This journal is © The Royal Society of Chemistry 2025 |
Click here to see how this site uses Cookies. View our privacy policy here.