DOI:
10.1039/D4QM00773E
(Research Article)
Mater. Chem. Front., 2025,
9, 100-108
A smart spiropyran-containing cellulose material for photopatterning, temperature and humidity sensing†
Received
6th September 2024
, Accepted 30th October 2024
First published on 30th October 2024
Abstract
Based on their stimuli-responsiveness, smart materials are able to undergo controllable physicochemical changes. As compared to the responsiveness to one specific stimulus, multiple stimuli-responsiveness would make smart materials adaptable to diverse environments, which is highly desired in the design of smart materials but appreciably more difficult to realize. Herein, an ammonium surfactant (SPA) based on spiropyran is designed for complexing with carboxymethylcellulose through an electrostatic route, affording a soft cellulose material (CMC–SPA) in solvent-free conditions. Thanks to the molecular design of SPA and the anisotropic arrangement of cellulose on SPA molecules, CMC–SPA exhibits triple stimuli-responsiveness by responding to light, heat and humidity. With good thermodynamic stabilities of different color states, CMC–SPA could well record optical information by changing colors under UV and visible irradiations. More interestingly, linear relationships between UV-visible absorption and temperature/humidity are established, endowing CMC–SPA with the functions of recording ceiling temperatures in inaccessible scenarios and indicating real-time environmental humidity. This study provides a design strategy for fabricating multiple stimuli-responsive materials, affording a new route for gaining smart biomaterials from biomacromolecules.
Introduction
Taking advantage of their external stimuli-responsive property, smart materials can undergo desired physicochemical changes in controllable ways. The fabrication of such materials requires the assistance of a specific functional molecule/motif that can respond to external stimuli. For example, a light-responsive actuator converts light energy into sophisticated mechanical motions based on a photochemical-driven molecular motor.1 Photochromic materials record optical information via the light-induced isomerization of photochromic molecules.2,3 And biomass-derived microgels gain water collection ability in arid conditions with the assistance of hygroscopic salts.4 Most of the developed smart materials can only respond to one unique external stimulus rather than multiple stimuli, which guarantees fine property control,5–7 but meanwhile limits the application of one smart material in different conditions. As compared to single stimulus-responsive materials, more advanced functional materials would be the ones capable of responding to multiple stimuli. These materials, being “smarter”, would accommodate different environments by exhibiting different property changes. The development of multiple stimuli-responsive materials needs either integrating different functional molecules/motifs together or designing multiple stimuli-responsive molecules, of which the latter is more challenging but very attractive.
Cellulose is one of the most abundant natural resources. Without special functional motifs at the molecular level, pure cellulose hardly shows response to the widely used external stimuli including light, heat and humidity. In order to realize stimuli-responsive cellulose materials, various strategies for incorporating functional molecules/motifs into cellulose through covalent modification or processing technologies have been developed, for example, providing fluorescent8–13 and photochromic14,15 materials. It is worth noting that these cellulose materials work in solutions or solid state. In stark contrast, external stimuli-responsive soft materials based on cellulose are rarely reported in the absence of any solvent,16 due to the intrinsic nature of biomacromolecules.17 As compared to the solution and solid states, the mesophase in solvent-free conditions may enable materials to undergo more physicochemical changes under external stimuli,18 for example, which may support the multiple stimuli-responsiveness of cellulose materials. However, to the best of our knowledge, in solvent-free conditions there has been no such cellulose material discovered yet. The fabrication of mesophase biomaterials from biomacromolecules could be achieved by introducing a flexible component for suppressing crystallization.19 Based on this idea, proteins,17,20,21 nucleic acids18,22–26 and even lignin27 have been prepared as thermotropic liquid crystals in solvent-free conditions. We also found that soft DNA materials could be rendered light-responsive by complexing DNA with flexible azobenzene-containing surfactants.28,29 These works inspire us to develop a cellulose material in a soft mesophase state that can support multiple stimuli-responsiveness under different external stimuli.
Subjected to the external effects of light, heat, metal ions, etc., spiropyran undergoes molecular reconstruction between spiropyran (SP) and merocyanine (MC) forms through heterolytic Cspiro–O bond cleavage and reforming processes with simultaneously exhibiting distinct changes of color, conformation and charge state,30,31 which provides an ideal option for designing multiple stimuli-responsive materials.32 While, the difficult isomerization of spiropyran in solvent-free conditions should always be considered in the design of spiropyran-based materials, caused by the absence of sufficient free volume for SP ⇄ MC conversion.33–41 In our previous studies, attaching a linear alkyl chain to the indole nitrogen of spiropyran has turned out to be an effective method for facilitating the isomerization of solvent-free spiropyran materials,42,43 as this kind of molecular design can provide sufficient free volume for isomerization through decreasing the molecular packing density. Based on this principle, herein a spiropyran-containing ammonium surfactant (SPA) with high molecular flexibility is designed for complexing with carboxymethylcellulose (CMC) to afford a soft cellulose material (CMC–SPA) showing a triple stimuli-responsive property (Fig. 1). Such a smart cellulose material capable of responding to light, heat and humidity could be used as a new potential information recording material, with temperature and humidity indicating functions in biological and biomedical fields.
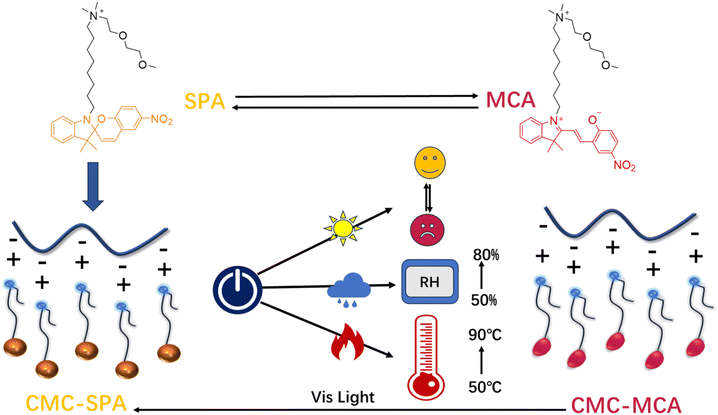 |
| Fig. 1 Chemical structure changes between SPA and MCA via ring-opening/ring-closing isomerization, and schematic representation of the triple external stimuli responses of CMC–SPA. For the ionic CMC–SPA complex, CMC is responsible for anisotropically arranging SPA, and SPA with high molecular flexibility can suppress the crystallization of CMC–SPA, which together provide a mesophase cellulose material with stimuli-responsiveness to light, heat and humidity. | |
Results and discussion
Synthesis and characterizations of SPA
CMC–SPA is an ionic complex composed of anionic CMC and cationic SPA, of which CMC was purchased and used directly and SPA was synthesized by us. In the molecular design of SPA, the C8 aliphatic chain and polyethylene glycol (PEG) chain can provide sufficient molecular flexibility for facilitating the isomerization of SPA under external stimuli and suppressing the crystallization of cellulose in solvent-free conditions. Meanwhile, the PEG chain also increases the hydrophilicity of SPA, giving the structural basis for realizing H2O-induced isomerization. SPA was prepared as a solid through firstly the one-pot synthesis of spiropyran motif44 and subsequently a quaternization with PEG–amine (Schemes S1 and S2, ESI†). The chemical structure of SPA was confirmed by NMR and HRMS (Fig. S1, ESI†).
In the solution state, the reversible SPA ⇄ MCA isomerization could be easily triggered by UV (365 nm, 32 mW cm−2) and visible (520 nm, 96 mW cm−2) light at room temperature (r.t.), exhibiting increased absorption at 450–650 nm from ring opening and decreased absorption from ring closure (Fig. S2, ESI†). To test the photoresponsive property in the solid state, SPA was prepared into a thin flake on a quartz glass slide and irradiated by UV/visible light. Under UV light, SPA can transform to MCA, showing an increased absorption peak at 450–700 nm (Fig. 2a), and visible light can induce the ring-closure isomerization to restore SPA, showing a reversed absorption intensity change (Fig. 2b). The SPA ⇄ MCA isomerization was accompanied by a distinct color change between dark-orange and red (Fig. 2c). The successful isomerization of solid-state SPA further proved that the molecular flexibility contributes to the photoresponsive property of spiropyran materials by decreasing the molecular packing density. While, as compared to the SPA solution, solid-state SPA exhibited a slower isomerization rate, which should be caused by the low light penetration efficiency of the solid sample and the intermolecular interactions between packed molecules. At r.t., MCA is the metastable isomer, which would thermodynamically transform back to SPA without UV treatment (Fig. S3 and S4, ESI†). MCA exhibited different half-lives in solution and solid state, being ca. 18 min and 1.6 h, respectively (Fig. 2d). The longer half-life in the solid state was caused by the π–π interaction and electrostatic force between MCA molecules. These intermolecular interactions could stabilize the MCA isomer.
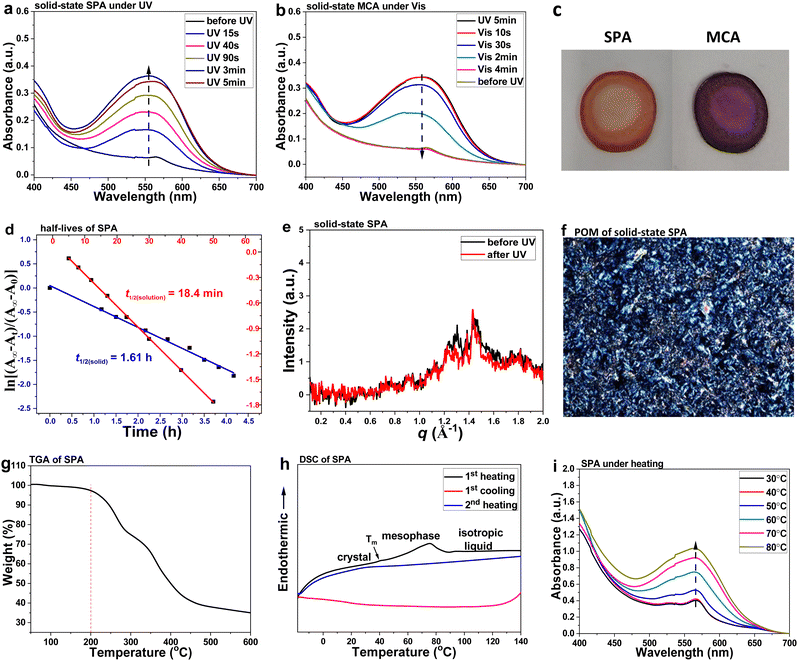 |
| Fig. 2 UV-visible absorption changes of solid-state (a) SPA under UV light and (b) MCA under visible light at r.t. The increased absorption peak under UV indicates the SPA-to-MCA isomerization, and the decreased absorption under visible light indicates the MCA-to-SPA isomerization. (c) Images of SPA and MCA at r.t. (d) Half-lives of MCA in solution and solid state at r.t. MCA exhibits a longer half-life in the solid state than in solution, due to the intermolecular interactions between planar and charged MCA molecules. (e) SAXS profile of SPA at r.t., showing a consistent ordered structure before and after UV irradiation. (f) POM image of SPA at r.t., indicating the ordered structure of SPA by showing focal-conic birefringence. Scale bar: 100 μm. (g) TGA profile of SPA, showing thermal integrity higher than 200 °C. (h) DSC profile of SPA, indicating the phase transitions of crystal-mesophase-isotropic liquid. Heating/cooling rate: 5 °C min−1. (i) Temperature-dependent UV-visible absorption changes of solid-state SPA, indicating a gradual SPA-to-MCA isomerization occurring when heating up to 40 °C. | |
Solid-state SPA is in an ordered structure at r.t., indicated by the diffraction peaks of the small-angle X-ray scattering (SAXS) profile (Fig. 2e), which could be well maintained under UV irradiation. The ordered structure was further confirmed by the focal-conic birefringence from polarized optical microscopy (POM) analysis (Fig. 2f). The thermal properties of SPA were investigated by thermogravimetric analysis (TGA) and differential scanning calorimetry (DSC). TGA indicated a thermal stability of SPA up to 200 °C (Fig. 2g). According to the first heating profile of DSC, SPA underwent a crystal-mesophase transition at 40 °C and a gradual mesophase-isotropic liquid transition at 45–90 °C (Fig. 2h). The weak endothermic peak from the melting process would result from the relatively loose packing density of SPA owing to its spiro conformation. While, when heating above 40 °C, the SPA-to-MCA isomerization gradually occurred (Fig. 2i), leading to the increased intermolecular interactions of π–π interaction and electrostatic force between planar MCA molecules. This resulted in the broad endothermic peak for the mesophase-isotropic liquid transition. The formation of isotropic liquid was confirmed by the temperature-dependent POM analysis (Fig. S5, ESI†). An immediate recrystallization of SPA on cooling to r.t. was difficult, evidenced by the disappearance of the exothermic peak from the cooling profile of DSC, but POM analysis showed a weak birefringence that indicates the ordered structure was forming (Fig. S5, ESI†).
Synthesis and characterizations of CMC–SPA
In this work, the used CMC is a commercially available polysaccharide with each monosaccharide unit assumingly carrying one negative charge, which provides a good “skeleton” structure for ionic complexation with SPA. Meanwhile, the anionic charge of CMC can also arrange SPA molecules along the polysaccharide chain, gaining sufficient free volume for SPA–MCA isomerization. The ionic CMC–SPA complex was prepared in a charge ratio of 1
:
1 through the electrostatic complexation between CMC and SPA in aqueous solution, followed by purifications via centrifugation and lyophilization, which afforded a soft material.
The TGA measurement showed that the resulting CMC–SPA ionic complex contains less than 3% water and has thermal integrity up to 200 °C (Fig. 3a). At r.t., CMC–SPA is in an ordered smectic phase with d-spacing distance of 3.90 nm, according to the SAXS analysis, showing a first diffraction peak at 0.16 Å−1 and the following harmonic peaks (Fig. 3b). Under heating condition, CMC–SPA in the mesophase could gradually transform to an isotropic liquid (Fig. 3c). The formation of the isotropic state was further confirmed by temperature-dependent POM analysis (Fig. 3d). After cooling to r.t., the ordered structure could be recovered in ∼5 h, indicating its thermotropic liquid crystalline property. The photoresponsive property of SPA was well preserved after complexing with CMC. CMC–SPA showed increased UV-visible absorption at 475–675 nm under UV irradiation at r.t. (Fig. 3e), and visible light induced a reversed absorption intensity change (Fig. 3f), indicating the SPA ⇄ MCA isomerization of the CMC–SPA complex. The photoinduced isomerization also caused a distinct color change (Fig. 3e-inset, Fig. 3f-inset). Interestingly, MCA exhibited a much improved stability after complexing with CMC. According to the recorded UV-visible absorption changes, the thermodynamic MCA-to-SPA isomerization in the cellulose complex at r.t. was significantly slowed down (Fig. 3g), presenting a half-life as long as 27 h (Fig. S6, ESI†). In CMC-MCA, CMC could provide an ionic environment, favoring the presence of zwitterionic MCA. Meanwhile, the anisotropic function of CMC on the arrangement of MCA molecules can also intensify the intermolecular interactions,45,46 which contribute to the extended half-life of MCA as well.
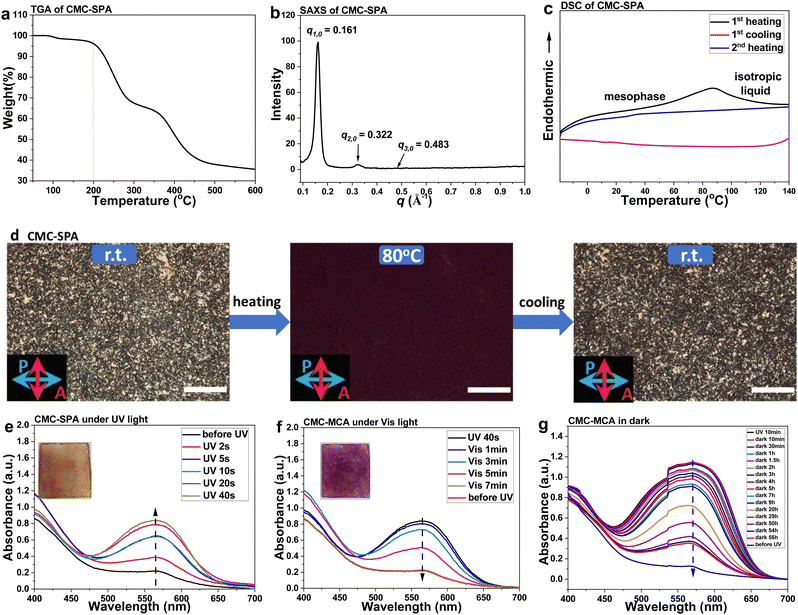 |
| Fig. 3 (a) TGA profile of CMC–SPA, indicating <3% water content and >200 °C thermal stability. (b) SAXS profile of CMC–SPA at r.t., indicating a lamellar structure. (c) DSC profiles of CMC–SPA, showing the gradual phase transition from mesophase to isotropic liquid under heating condition. Heating/cooling rate: 5 °C min−1. (d) Temperature-dependent POM analysis of CMC–SPA, indicating the thermotropic liquid crystal property of CMC–SPA by showing disappearance of birefringence at 80 °C and reappearance of birefringence after cooling to r.t. Scale bar: 50 μm. UV-visible absorption changes of solvent-free (e) CMC–SPA under UV light and (f) CMC–MCA under visible light at r.t., indicating the reversible SPA–MCA isomerization in the ionic complex. Insets: Images of CMC–SPA and CMC–MCA samples at r.t. (g) The temporal evolved UV-visible absorption spectral changes of CMC–MCA in dark condition at r.t., presenting a much slower thermodynamic MCA-to-SPA isomerization. | |
Responses of CMC–SPA to heat and humidity
Subsequently, we investigated the response of CMC–SPA to heat. A thin flake sample with 9 μm thickness was prepared on a quartz glass slide for the tests (Fig. S7, ESI†). The transition of stable SPA to metastable MCA could be realized by heat-induced ring-opening isomerization, this endothermic process requiring sufficient heat energy. The temperature-dependent UV-visible absorption spectral changes of CMC–SPA indicated that negligible isomerization occurred when the temperature was below 50 °C by showing almost no absorption intensity increase (Fig. 4b). Upon heating the sample to higher than 50 °C, continuous ring-opening isomerization of SPA would happen. According to the temporal evolved UV-visible absorption changes at 50 °C (Fig. S8, ESI†), a heating incubation time of 5 min is sufficient (Fig. 4a). Therefore, in three repeating groups, we heated the flake sample for 5 min at 50 °C, 60 °C, 70 °C, 80 °C and 90 °C, and recorded the UV-visible absorption changes caused by the temperature changes (Fig. 4b and Fig. S9, ESI†). With the temperature increasing, the absorption of CMC–SPA was intensified due to the heat-induced ring-opening reaction. Interestingly, the absorption intensity change at 560 nm of CMC–SPA showed a linear relationship with the temperature change (Fig. 4c). While, when the temperature was higher than 90 °C, no linear relationship between UV-visible absorption intensity and temperature was found, due to the sample thickness change that was caused by the formation of the isotropic liquid state (Fig. 3c). Based on the ring-opening isomer of MCA gaining a long half-life in the ionic complex (Fig. 3g), CMC–SPA could be a temperature-indicating material used for recording the ceiling temperature in some specific scenarios, for example, in inaccessible environments.
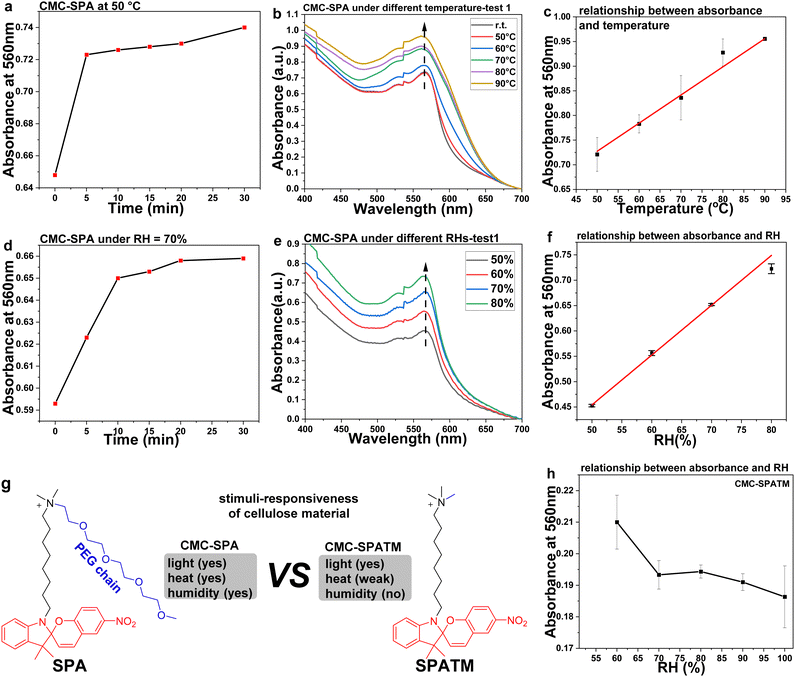 |
| Fig. 4 (a) Plot of UV-visible absorption changes at 560 nm of CMC–SPA at 50 °C within 30 min, indicating that CMC–SPA requires 5 min incubation for reaching a stable state. (b) Temperature-dependent UV-visible absorption changes of CMC–SPA, showing increasing SPA-to-MCA isomerization with temperature increasing. (c) Plot of absorption changes at 560 nm of CMC–SPA at different temperatures, showing a linear relationship between absorbance and temperature. (d) Plot of absorption changes at 560 nm of CMC–SPA under RH = 70% within 30 min, indicating that CMC–SPA requires 10 min incubation for reaching a stable state. (e) Humidity-dependent UV-visible absorption changes of CMC–SPA at r.t., showing the increasing SPA-to-MCA isomerization with RH increasing. (f) Plot of absorption changes at 560 nm of CMC–SPA at different RHs, showing a linear relationship between absorbance and RH value. (g) Comparisons of the chemical structures and resulting physicochemical properties between SPA and SPATM. (h) Plot of absorption changes at 560 nm of CMC–SPATM at different RHs, showing a general decreasing trend of the absorption intensity with RH increasing. This phenomenon could be caused by the dissolution process of solid-state CMC–SPATM under high RHs, which induced a sample thickness change. | |
A thin flake was also prepared for investigating the humidity-induced ring-opening reaction of CMC–SPA at r.t. Initially, the UV-visible absorption changes of CMC–SPA at relative humidity (RH) = 70% were monitored (Fig. S10, ESI†). In 10 min, the absorption intensity at 560 nm showed a fast increase and then reached a plateau (Fig. 4d), indicating that a 10 min incubation of CMC–SPA under specific RH condition was necessary. Then, also in three repeat testing groups, CMC–SPA was incubated for 10 min at RH = 50%, 60%, 70%, 80%, 90% and 100%, which afforded the RH-induced UV-visible absorption changes at r.t. (Fig. 4e and Fig. S11, ESI†). A linear relationship between absorption intensity change at 560 nm of CMC–SPA and RH value ranging from 50% to 80% was observed (Fig. 4f). When RH was higher than 80%, CMC–SPA could not reflect the exact humidity change, showing an irregular UV-visible absorption intensity change (Fig. S12a–c, ESI†), probably due to the fact that high RH > 80% induced a film thickness change by absorbing too much water. In contrast, a very weak UV-visible absorption intensity increase was observed on subjecting CMC–SPA to RH = 20–40% (Fig. S12d, ESI†), indicating that a relatively dry condition could only induce weak isomerization with RH < 50%. Therefore, CMC–SPA could be developed into a hygrometer material with an accurate indication for the real-time RH at 50–80% and a rough estimation in humid conditions with RH > 80% or <50%.
In order to gain insight into the triple stimuli-responsive property of CMC–SPA to light, heat and humidity, a control cellulose material, CMC–SPATM, was fabricated by electrostatically complexing CMC with surfactant SPATM, applying the same synthetic method as that for CMC–SPA. SPATM has a similar chemical structure to SPA but without the PEG chain (Fig. 4g). In the molecular design of SPA, the presence of the PEG chain provides both hydrophilicity and molecular flexibility, which contribute to the preparation of CMC–SPA in soft mesophase state and high synthetic yield. As aqueous solutions were used for the materials’ preparation, a high water solubility of SPA could improve the concentration of SPA in water and thus favor a high synthetic yield. In contrast to SPA, after complexing with CMC, SPATM provided CMC–SPATM as a brittle solid material due to the low molecular flexibility (Fig. S13, ESI†). In the stimuli-responsive property tests, CMC–SPATM exhibited a reversible photochromic property under UV/visible irradiations (Fig. S14, ESI†). Meanwhile, the response of CMC–SPATM became weak and showed no linear relationship between absorbance and temperature under the same testing temperature range as that used for CMC–SPA (Fig. S15 and S16, ESI†), which was caused by the higher molecular packing density of SPATM sublayer in CMC–SPATM due to the low molecular flexibility of SPATM. The absence of the PEG chain also weakened the hydrophilicity of SPATM in the corresponding cellulose material, leading to no detectable humidity-induced isomerization but showing a general decreasing trend of the absorption intensity with RH increasing (Fig. 4h and Fig. S17, S18, ESI†). This would be induced by the sample thickness change during the “dissolving” of solid-state CMC–SPATM under highly humid conditions.
As compared to CMC–SPATM, CMC–SPA is a smarter cellulose material that can respond to light, heat and humidity (Fig. 4g). Such a triple stimuli-responsive property is generated from the used surfactant SPA and the anisotropic arrangement of CMC on SPA molecules. CMC–SPA is a typical thermotropic liquid crystal material whose responsive property is determined by the mobility part from changing configuration.19 In our work, the successful isomerization of 6-nitrospiropyran is the structural basis for cellulose materials gaining responsiveness to external stimuli. Besides the C8 chain, SPA contains one more PEG chain that provides sufficient molecular flexibility for SP-MC isomerization of 6-nitrospiropyran by effectively reducing the packing density of the SPA sublayer. As a result, CMC–SPA could respond to heat more efficiently than CMC–SPATM. Furthermore, the presence of PEG also increases the exposure probability of 6-nitrospiropyran to water in the SPA sublayer of CMC–SPA, producing the humidity response of CMC–SPA. Meanwhile, SPATM with no PEG chain has less molecular flexibility and hydrophobicity, leading to a higher packing density in the surfactant sublayer of CMC–SPATM than that of CMC–SPA. With such a highly packed structure, CMC–SPATM exhibited poor or no responsiveness to heat and humidity. And the different chemical structures of SPA and SPATM also result in different physical states, as CMC–SPA presents as a soft mesophase material and CMC–SPATM is in the solid state.
Application tests with CMC–SPA
The triple stimuli-responsive property enables CMC–SPA to be applicable in various scenarios, for example, being an information recording material, and a temperature- and humidity-indicating material. To verify its information recording ability, a thin layer of CMC–SPA was attached to a glass slide and covered by different aluminum foil masks (Fig. 5a). After being irradiated by UV light, different patterns of snowflake, heart and sun could be well recorded by CMC–SPA, which could also be erased quickly by visible light. The long half-life of CMC–SPA (26 h from Fig. 3g and Fig. S6, ESI†) would guarantee the information safe before next use. All of these attributes would render CMC–SPA as an excellent information recording biomaterial for recycling use.
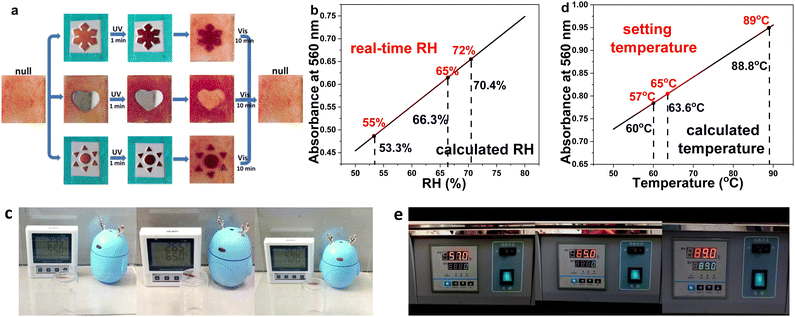 |
| Fig. 5 (a) Optical information recording tests with CMC–SPA, showing the fast, stable and erasable photopatterning ability of CMC–SPA. (b) Humidity indicating tests by utilizing the humidity-responsive property of CMC–SPA. The calculated RH values from the linear fit between absorbance and RH could accurately indicate the real-time RH. (c) Images of hydrometer with real-time RH values. (d) Temperature indicating tests by utilizing the heat-responsive property of CMC–SPA. The calculated temperatures from the linear fit between absorbance and temperature could accurately record the ceiling temperatures in an inaccessible oven. (e) Images of oven with setting temperatures. | |
Taking advantage of the linear relationship between absorbance and RH (Fig. 4f), CMC–SPA can serve as a hydrometer for indicating the real-time RH. We put CMC–SPA under three different RH conditions for 10 min, and then measured the UV-visible absorptions (Fig. S19, ESI†). By fitting the absorption intensity at 560 nm of each test to the linear function, RH = 53.3%, 66.3% and 70.4% could be calculated (Fig. 5b), respectively, which accurately indicated the actual RH = 55%, 65% and 72% (Fig. 5c), showing an error of RH difference <2%. CMC–SPA could also be a good heat indicator for recording the ceiling temperature in some inaccessible scenarios. For example, we put the CMC–SPA sample into an oven, and set the temperature at each of 57 °C, 65 °C and 89 °C. After reaching the setting temperatures, the oven was shut down and allowed to cool to r.t. Then, the sample was taken out of the oven and measured using a UV-visible absorption instrument. By fitting to the linear function between absorbance and temperature (Fig. 4c), calculated temperatures of 60 °C, 63.6 °C and 88.8 °C were be obtained (Fig. 5d), which accurately reflected and recorded the maximum temperatures in the heating tests (Fig. 5e). This kind of temperature memory material would be important for monitoring the temperature change in isolated environments.
Conclusions
In this study, a spiropyran-containing ammonium surfactant SPA was designed for electrostatically complexing with CMC to provide a soft biomaterial: CMC–SPA. Thanks to the molecular design and anisotropic arrangement of CMC on SPA, SPA could isomerize by responding to light, heat and humidity in the ionic complex, leading to CMC–SPA being a triple stimuli-responsive material. In the ionic complex, the metastable MCA has a half-life of over 26 h, which favors stable information recording. Taking advantage of the responsiveness to light, CMC–SPA exhibits good photopatterning ability. More interestingly, linear relationships between UV-visible absorbance and temperature/RH were well established, endowing CMC–SPA with the functions of recording ceiling temperatures in inaccessible scenarios and indicating real-time RH for the environment. This study provides a design strategy for fabricating multiple stimuli-responsive materials, affording a way of gaining smart biomaterials from biomacromolecules.
Data availability
The data supporting this article have been included as part of the ESI.†
Conflicts of interest
There are no conflicts to declare.
Acknowledgements
This work was supported by the Natural Science Foundation of Shandong Province (no. ZR2023MB104), National Natural Science Foundation of China (no. 21805150) and Starting Grant from Qingdao University of Science and Technology (no. 12030430010723).
References
- J. Hou, A. Mondal, G. Long, L. de Haan, W. Zhao, G. Zhou, D. Liu, D. J. Broer, J. Chen and B. L. Feringa, Photo-responsive Helical Motion by Light-Driven Molecular Motors in a Liquid-Crystal Network, Angew. Chem., Int. Ed., 2021, 60, 8251–8257 CrossRef CAS PubMed.
- Y. Yang, J. He, Z. He and G. Jiang, Enhancement of Solid-State Reversible Photochromism by Incorporation of Rigid Steric Hindrance Groups, Adv. Opt. Mater., 2021, 9, 2001584 CrossRef CAS.
- Q. Qi, C. Li, X. Liu, S. Jiang, Z. Xu, R. Lee, M. Zhu, B. Xu and W. Tian, Solid-State Photoinduced Luminescence Switch for Advanced Anticounterfeiting and Super-Resolution Imaging Applications, J. Am. Chem. Soc., 2017, 139, 16036–16039 CrossRef CAS.
- W. Guan, C. Lei, Y. Guo, W. Shi and G. Yu, Hygroscopic Microgels-Enabled Rapid Water Extraction from Arid Air, Adv. Mater., 2022, 2207786 Search PubMed.
- Y. Wang, Z. Chen, J. Li, Y. Wen, J. Li, Y. Lv, Z. Pei and Y. Pei, A Paramagnetic Metal-Organic Framework Enhances Mild Magnetic Hyperthermia Therapy by Downregulating Heat Shock Proteins and Promoting Ferroptosis via Aggravation of Two-Way Regulated Redox Dyshomeostasis, Adv. Sci., 2024, 11, 2306178 CrossRef CAS.
- J. Li, B. Hu, Z. Chen, J. Li, W. Jin, Y. Wang, Y. Wan, Y. Lv, Y. Pei, H. Liu and Z. Pei, Mn(III)-mediated carbon-centered radicals generate an enhanced immunotherapeutic effect, Chem. Sci., 2024, 15, 765–777 RSC.
- W. Jin, Z. Chen, Y. Wang, J. Li, J. Li, Y. Pei and Z. Pei, Nano metal-photosensitizer based on Aza-BODIPY-Cu complex for CDT-enhanced dual phototherapy, Chin. Chem. Lett., 2024, 35, 109328 CrossRef CAS.
- H. Nawaz, X. Zhang, S. Chen, T. You and F. Xu, Recent studies on cellulose-based fluorescent smart materials and their applications: a comprehensive review, Carbohydr. Polym., 2021, 267, 118135 CrossRef CAS.
- J. Fan, S. Zhang, F. Li and J. Shi, Cellulose-based sensors for metal ions detection, Cellulose, 2020, 27, 5477–5507 CrossRef CAS.
- Z. Zhang, G. Liu, X. Li, S. Zhang, X. Lü and Y. Wang, Design and Synthesis of Fluorescent Nanocelluloses for Sensing and Bioimaging Applications, ChemPlusChem, 2020, 85, 487–502 CrossRef CAS PubMed.
- X. Chen, W. Wu, L. Liu, J. Hao and S. Dong, DNA-involved thermotropic liquid crystals from catanionic vesicles, Colloids Surf., A, 2022, 641, 128607 CrossRef CAS.
- Q. Wang, J. Cai, K. Chen, X. Liu and L. Zhang, Construction of Fluorescent Cellulose Biobased Plastics and their Potential Application in Anti-Counterfeiting Banknotes, Macromol. Mater. Eng., 2016, 301, 377–382 CrossRef CAS.
- M. Li, Z. Liu, S. Wang, D. G. Calatayud, W.-H. Zhu, T. D. James, L. Wang, B. Mao and H.-N. Xiao, Fluorescence detection and removal of copper from water using a biobased and biodegradable 2D soft material, Chem. Commun., 2018, 54, 184–187 RSC.
- A. Abdollahi, A. Mouraki, M. H. Sharifian and A. R. Mahdavian, Photochromic properties of stimuli-responsive cellulosic papers modified by spiropyran-acrylic copolymer in reusable pH-sensors, Carbohydr. Polym., 2018, 200, 583–594 CrossRef CAS PubMed.
- A. Abdollahi, A. Herizchi, H. Roghani-Mamaqani and H. Alidaei-Sharif, Interaction of photoswitchable nanoparticles with cellulosic materials for anticounterfeiting and authentication security documents, Carbohydr. Polym., 2020, 230, 115603 CrossRef CAS.
- L. Zhang, C. Zhang, C. Xie and Z. Wu, Fluorescent solvent-free cellulose ionic complex towards thermostable luminescent coating material, Dyes Pigm., 2022, 206, 110611 CrossRef CAS.
- A. W. Perriman, H. Cölfen, R. W. Hughes, C. L. Barrie and S. Mann, Solvent-Free Protein Liquids and Liquid Crystals, Angew. Chem., Int. Ed., 2009, 48, 6242–6246 CrossRef CAS.
- K. Liu, D. Chen, A. Marcozzi, L. Zheng, J. Su, D. Pesce, W. Zajaczkowski, A. Kolbe, W. Pisula, K. Müllen, N. A. Clark and A. Herrmann, Thermotropic liquid crystals from biomacromolecules, Proc. Natl. Acad. Sci. U. S. A., 2014, 111, 18596 CrossRef CAS.
- C. Tschierske, Liquid crystal engineering – new complex mesophase structures and their relations to polymer morphologies, nanoscale patterning and crystal engineering, Chem. Soc. Rev., 2007, 36, 1930–1970 RSC.
- K. Liu, D. Pesce, C. Ma, M. Tuchband, M. Shuai, D. Chen, J. Su, Q. Liu, J. Y. Gerasimov, A. Kolbe, W. Zajaczkowski, W. Pisula, K. Müllen, N. A. Clark and A. Herrmann, Solvent-Free Liquid Crystals and Liquids Based on Genetically Engineered Supercharged Polypeptides with High Elasticity, Adv. Mater., 2015, 27, 2459–2465 CrossRef CAS PubMed.
- J. Sun, C. Ma, S. Maity, F. Wang, Y. Zhou, G. Portale, R. Göstl, W. H. Roos, H. Zhang, K. Liu and A. Herrmann, Reversibly Photo-Modulating Mechanical Stiffness and Toughness of Bioengineered Protein Fibers, Angew. Chem., Int. Ed., 2021, 60, 3222–3228 CrossRef CAS PubMed.
- K. Liu, M. Shuai, D. Chen, M. Tuchband, J. Y. Gerasimov, J. Su, Q. Liu, W. Zajaczkowski, W. Pisula, K. Müllen, N. A. Clark and A. Herrmann, Solvent-free Liquid Crystals and Liquids from DNA, Chem. – Eur. J., 2015, 21, 4898–4903 CrossRef CAS PubMed.
- L. Zhang, Y. Qu, J. Gu, H. Liu, Z. Wu and X. Luo, Powerful tailoring effects of counterions of ammonium surfactants on the phase transitions of solvent-free DNA thermotropic liquid crystals, J. Mol. Liq., 2021, 337, 116480 CrossRef CAS.
- G. D. Han, S. S. Park, Y. Liu, D. Zhitomirsky, E. Cho, M. Dincă and J. C. Grossman, Photon energy storage materials with high energy densities based on diacetylene–azobenzene derivatives, J. Mater. Chem. A, 2016, 4, 16157–16165 RSC.
- L. Xu, M. Chen and J. Hao, Ferrofluids of Thermotropic Liquid Crystals by DNA–Lipid Hybrids, J. Phys. Chem. B, 2017, 121, 420–425 CrossRef CAS PubMed.
- Z. Meng, Q. Liu, Y. Zhang, J. Sun, C. Yang, H. Li, M. Loznik, R. Göstl, D. Chen, F. Wang, N. A. Clark, H. Zhang, A. Herrmann and K. Liu, Highly Stiff and Stretchable DNA Liquid Crystalline Organogels with Super Plasticity, Ultrafast Self-Healing, and Magnetic Response Behaviors, Adv. Mater., 2022, 34, 2106208 CrossRef CAS PubMed.
- L. Zhang, C. Zhang, K. Wang, J. Liu, C. Xie and Z. Wu, Fluorescent solvent-free lignin ionic complexes with thermostability toward a luminescent hydrophobic coating material, Mater. Chem. Front., 2022, 6, 2122–2127 RSC.
- L. Zhang, S. Maity, K. Liu, Q. Liu, R. Göstl, G. Portale, W. H. Roos and A. Herrmann, Nematic DNA Thermotropic Liquid Crystals with Photoresponsive Mechanical Properties, Small, 2017, 13, 1701207 CrossRef.
- L. Zhang, Y. Qu, J. Gu, Y. Liu, Z. Tang, C. Zhang, H. Liu, J. Liu, Z. Wu and X. Luo, Photoswitchable solvent-free DNA thermotropic liquid crystals toward self-erasable shape information recording biomaterials, Mater. Today Bio, 2021, 12, 100140 CrossRef CAS PubMed.
- V. I. Minkin, Photo-, Thermo-, Solvato-, and Electrochromic Spiroheterocyclic Compounds, Chem. Rev., 2004, 104, 2751–2776 CrossRef CAS.
- R. Klajn, Spiropyran-based dynamic materials, Chem. Soc. Rev., 2014, 43, 148–184 RSC.
- S. Mo, Q. Meng, S. Wan, Z. Su, H. Yan, B. Z. Tang and M. Yin, Tunable Mechanoresponsive Self-Assembly of an Amide-Linked Dyad with Dual Sensitivity of Photochromism and Mechanochromism, Adv. Funct. Mater., 2017, 27, 1701210 CrossRef.
- J. Harada, Y. Kawazoe and K. Ogawa, Photochromism of spiropyrans and spirooxazines in the solid state: low temperature enhances photocoloration, Chem. Commun., 2010, 46, 2593–2595 RSC.
- S. Bénard and P. Yu, A spirooxazine showing crystalline state photochromism, Chem. Commun., 2000, 65–66 RSC.
- S. Bénard and P. Yu, New Spiropyrans Showing Crystalline-State Photochromism, Adv. Mater., 2000, 12, 48–50 CrossRef.
- D. G. Patel, J. B. Benedict, R. A. Kopelman and N. L. Frank, Photochromism of a spirooxazine in the single crystalline phase, Chem. Commun., 2005, 2208–2210 RSC.
- Y. Yang, J. He, Z. He and G. Jiang, Enhancement of Solid-State Reversible Photochromism by Incorporation of Rigid Steric Hindrance Groups, Adv. Opt. Mater., 2020, 2001584 Search PubMed.
- Y. Zhang, J. Yu, J. Wang, N. J. Hanne, Z. Cui, C. Qian, C. Wang, H. Xin, J. H. Cole, C. M. Gallippi, Y. Zhu and Z. Gu, Thrombin-Responsive Transcutaneous Patch for Auto-Anticoagulant Regulation, Adv. Mater., 2017, 29, 1604043 CrossRef.
- L. Wu, R. Chen, Z. Luo and P. Wang, Solid-state photochromism and acidochromism multifunctional materials constructed by tetraphenylethene and spiropyran, J. Mater. Sci., 2020, 55, 12826–12835 CrossRef CAS.
- B. Fang, M. Chu, L. Tan, P. Li, Y. Hou, Y. Shi, Y. S. Zhao and M. Yin, Near-Infrared Microlasers from Self-Assembled Spiropyrane-Based Microsphercial Caps, ACS Appl. Mater. Interfaces, 2019, 11, 38226–38231 CrossRef CAS PubMed.
- B. Lv, Z. Wu, C. Ji, W. Yang, D. Yan and M. Yin, Spiropyran-induced one-dimensional cyclodextrin microcrystals with light-driven fluorescence change, J. Mater. Chem. C, 2015, 3, 8519–8525 RSC.
- L. Zhang, Y. Deng, Y. Tang, C. Xie and Z. Wu, Solid-state spiropyrans exhibiting photochromic properties based on molecular flexibility, Mater. Chem. Front., 2021, 5, 3119–3124 RSC.
- Y. Deng, L. Zhang, C. Zhang, J. Gu, J. Liu, H. Liu, C. Xie and Z. Wu, Tailoring effects of the chain length and terminal substituent on the photochromism of solid-state spiropyrans, Org. Biomol. Chem., 2021, 19, 8722–8726 RSC.
- L. Zhang, Y. Deng, Z. Tang, N. Zheng, C. Zhang, C. Xie and Z. Wu, One-Pot Synthesis of Spiropyrans, Asian J. Org. Chem., 2019, 8, 1866–1869 CrossRef CAS.
- W. Luo, Y. Feng, C. Cao, M. Li, E. Liu, S. Li, C. Qin, W. Hu and W. Feng, A high energy density azobenzene/graphene hybrid: a nano-templated platform for solar thermal storage, J. Mater. Chem. A, 2015, 3, 11787–11795 RSC.
- W. Luo, Y. Feng, C. Qin, M. Li, S. Li, C. Cao, P. Long, E. Liu, W. Hu, K. Yoshino and W. Feng, High-energy, stable and recycled molecular solar thermal storage materials using AZO/graphene hybrids by optimizing hydrogen bonds, Nanoscale, 2015, 7, 16214–16221 RSC.
|
This journal is © the Partner Organisations 2025 |
Click here to see how this site uses Cookies. View our privacy policy here.