DOI:
10.1039/D4QO01570C
(Research Article)
Org. Chem. Front., 2025,
12, 136-141
Palladium-catalyzed synthesis of indene-1-acetates via sequential double carbopalladation and aryloxycarbonylation†
Received
24th August 2024
, Accepted 26th October 2024
First published on 28th October 2024
Abstract
A novel palladium-catalyzed cascade reaction of o-iodostyrenes, internal alkynes and formates to access various indene-1-acetates was developed. This method enables the construction of three C–C bonds and one C–O bond through sequential double carbopalladation and aryloxycarbonylation under a palladium/air system. This protocol exhibits high regioselectivity and wide functional group tolerance. In addition, the synthetic utility of this protocol has been successfully demonstrated by the gram-scale synthesis and the late-stage modification of a series of complex bioactive molecules.
Introduction
Indene derivatives have gained widespread attention in medicinal chemistry, organic chemistry and materials science due to their unique bioactive, chemical and physical properties.1 In this context, some efficient methods for their construction have been developed, and typical strategies include nucleophilic-attack-induced cyclizations,2 intramolecular electrophilic substitution reactions,3 ring expansion and contraction,4 and transition-metal-catalyzed cyclizations.5 Among them, palladium-catalyzed cross-coupling annulation has emerged as a powerful tool for accessing indene derivatives.6 For example, Feng and co-workers developed a practical synthetic route for the construction of highly functionalized 1-trifluoromethyl-1H-indenes by a fluoride nucleophilic-addition-induced [3 + 2] annulation strategy (Scheme 1a).6a Nevertheless, the existing methods still suffer from a limited substrate scope and poor product diversity. The examples are quite rare, especially for introducing different types of functional groups into indene skeletons. Thus it would be highly desirable to exploit new and efficient methods with a broad substrate scope for the assembly of functionalized indene derivatives.
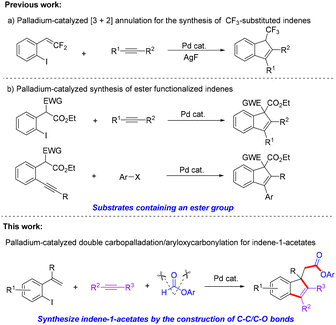 |
| Scheme 1 Synthesis of functionalized indene derivatives. | |
On the other hand, despite the fact that Larock and Liang have made an impressive breakthrough to achieve ester functionalized indenes via a directed palladium-catalyzed strategy involving substrates containing an ester group (Scheme 1b),7 so far, protocols employing a CO surrogate as a carbonyl source for the synthesis of ester functionalized indene derivatives have not been reported. Aryloxycarbonylative reactions have become one of the most straightforward and facile methods to synthesize valuable ester compounds.8 Usually, palladium-catalyzed aryloxycarbonylation often utilizes CO and alcohols to trap palladium intermediates for the synthesis of esters.9 Considering the high toxicity and flammability of CO gas, a variety of CO surrogates, such as TFBen,10N-formylsaccharin,11 COCl2,12 formates,13 and M(CO)6,14 have been developed to avoid the direct use of CO. On the basis of our recent work15 and our ongoing interest in palladium-catalyzed cascade cyclization and aryloxycarbonylation,16 we herein report a new Pd-catalyzed three-component protocol for the direct synthesis of indene-1-acetates through a tandem double carbopalladation and subsequent aryloxycarbonylation with formates as CO surrogates (Scheme 1c). This reaction provides a versatile and simple platform for the construction of densely substituted indene-1-acetates that would otherwise not be easily accessed using the already reported methods. Importantly, this research is essentially an assembly process consisting of four components.
Results and discussion
Our initial investigation focused on the three-component cascade reaction of 1-iodo-2-(prop-1-en-2-yl)benzene (1a), 1,2-diphenylethyne (2a) and phenyl formate (3a). After an extensive screening of the reaction parameters, it was found that Pd(MeCN)2Cl2, XPhos and K2CO3 in DME at 70 °C under an air atmosphere for 12 h were the optimal conditions for this conversion (Table 1, entry 1), affording the desired 4a in a yield of 61%. Other palladium catalysts, such as Pd(OAc)2, [Pd (C3H5)Cl]2, Pd2(dba)3 and PdCl2, proved to be less efficient than PdCl2(MeCN)2 in terms of yield (entries 2–5). Then it was found that other phosphine ligands could not promote this three-component reaction (entries 6–10). The examination of other bases, including KOAc, KOMe, K3PO4 and Et3N, indicated that all of them were inferior to K2CO3 (entries 11–14). We next screened the effect of solvents and found that DCE and MeCN provided inferior results (entries 15 and 16). The amount of solvent was found to be vital for the efficiency of the transformation. With 2 mL of DME, the yield of 4a was significantly diminished (entry 17). The change in reaction temperature resulted in a reduced yield (entries 18 and 19). Finally, the reaction atmosphere was investigated and an N2 atmosphere did not provide a better outcome (entry 20).
Table 1 Optimization of the reaction conditionsa

|
Entry |
Variation from the standard conditions |
Yieldb (%) |
Reaction conditions unless otherwise noted: 1a (0.2 mmol), 2a (0.4 mmol), 3a (0.6 mmol), Pd(MeCN)2Cl2 (10 mol%), XPhos (20 mol%), K2CO3 (0.6 mmol), DME (1.0 mL, 0.2 M), 70 °C, 12 h under air conditions.
Isolated yield.
Pd(MeCN)2Cl2 (5 mol%).
|
1 |
None |
61 (48c) |
2 |
Pd(OAc)2 instead of Pd(MeCN)2Cl2 |
40 |
3 |
[Pd(C3H5)Cl]2 instead of Pd(MeCN)2Cl2 |
47 |
4 |
Pd2(dba)3 instead of Pd(MeCN)2Cl2 |
56 |
5 |
PdCl2 instead of Pd(MeCN)2Cl2 |
54 |
6 |
BrettPhos as ligand |
51 |
7 |
P(p-FC6H4)3 as ligand |
16 |
8 |
SPhos as ligand |
33 |
9 |
Q-Phos as ligand |
39 |
10 |
Xantphos as ligand |
50 |
11 |
KOAc instead of K2CO3 |
57 |
12 |
KOMe instead of K2CO3 |
53 |
13 |
K3PO4 instead of K2CO3 |
32 |
14 |
Et3N instead of K2CO3 |
0 |
15 |
DCE as solvent |
55 |
16 |
MeCN as solvent |
46 |
17 |
DME as solvent (2 mL) |
40 |
18 |
80 °C instead of 70 °C |
59 |
19 |
60 °C instead of 70 °C |
54 |
20 |
Under an N2 atmosphere |
49 |
With the optimal reaction conditions in hand, the scope of this palladium-catalyzed double carbopalladation/aryloxycarbonylation reaction with regard to o-iodostyrenes 1 was examined (Scheme 2). Notably, o-iodostyrenes bearing a series of functional groups, including electron-donating groups (Me and OMe) and electron-withdrawing groups (F, Cl, and CF3), at the 5- or 4-position on the benzene ring were investigated, and the corresponding products 4b–4h were obtained in moderate yields. Gratifyingly, despite the presence of steric congestion, when a substituent such as methyl was introduced at the ortho-position of an iodide, a moderate yield (60%) of the indene product (4i) was obtained. Besides the methyl group, ethyl and butyl substituents on the olefins also reacted smoothly, providing the desired products (4j and 4k) in 50% and 48% yields, respectively. Finally, trisubstituted or monosubstituted olefins were not compatible with this reaction.
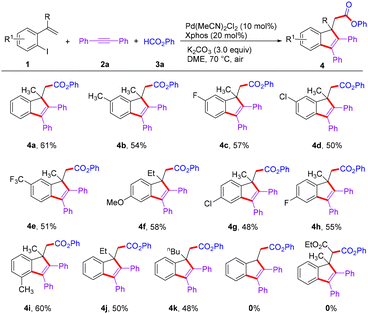 |
| Scheme 2 Substrate scope of o-iodostyrenes. Reaction conditions unless otherwise noted: 1 (0.2 mmol), 2a (0.4 mmol), 3a (0.6 mmol), Pd(MeCN)2Cl2 (10 mol%), XPhos (20 mol%), K2CO3 (0.6 mmol), DME (1.0 mL, 0.2 M), 70 °C, 12 h under air conditions. | |
Subsequently, a wide range of internal alkynes and formates were investigated by reaction with o-iodostyrene 1a (Scheme 3). First, symmetrical diaryl alkynes bearing different functional groups, ranging from electron-donating groups (Me, tBu, and OMe) to electron-withdrawing groups (F and CF3) on the aromatic rings at a para-position relative to the alkynyl group, were all compatible, giving products 4l–4p in 44–63% yields. The substituents (OMe, F, and Cl) at the meta-position on the aromatic rings of diaryl alkynes also afforded comparable results (4q–4s). Also, the reaction was successful with dialkyl alkynes, furnishing products 4t–4v in moderate yields. Significantly, unsymmetrical alkynes also displayed good reactivity, but a pair of regioisomers were observed (4w and 4w′). The scope of formates was then tested (Scheme 3). To our satisfaction, a series of aryl formats bearing diverse substituents (Me, OMe, F, Cl, and CF3) at any position of the benzene ring reacted efficiently to provide the target products 4x–4ag in 38–62% yields. Moreover, the bulky dimethoxyl substituent at the ortho-position on the aromatic ring of formate delivered product 4ah in 41% yield. Bicyclic substituted formates were well tolerated, furnishing the corresponding products 4ai and 4aj in yields of 54% and 46%, respectively. The structure of compound 4aj was further confirmed by X-ray crystallographic analysis.17
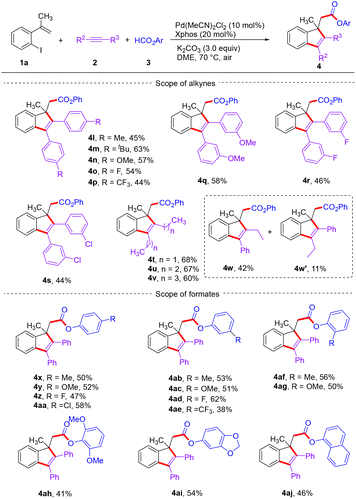 |
| Scheme 3 Substrate scope of alkynes and formates. Reaction conditions unless otherwise noted: 1a (0.2 mmol), 2 (0.4 mmol), 3 (0.6 mmol), Pd(MeCN)2Cl2 (10 mol%), XPhos (20 mol%), K2CO3 (0.6 mmol), DME (1.0 mL, 0.2 M), 70 °C, 12 h under air conditions. | |
Encouraged by the efficiency of this strategy, the implementation of the protocol for the late-stage modification of bioactive molecules was further investigated (Scheme 4). Formates derived from bioactive molecules, such as carvacrol, flavone, raspberry ketone, tocopherol, pterostilbene and estrone, were found to efficiently produce the corresponding products 5a–5f in moderate yields. Collectively, this approach indicated that the reactions reported herein have powerful application prospects in synthesizing medicinally relevant compounds.
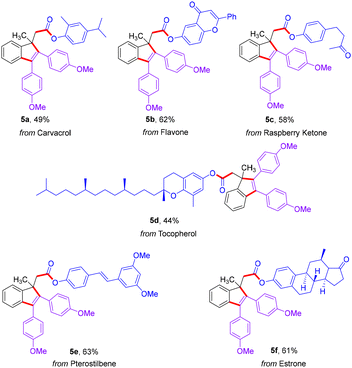 |
| Scheme 4 Late-stage modification of complex molecules. | |
To examine the synthetic potential of this reaction, a scale-up experiment was conducted under the standard conditions, resulting in the successful production of product 4a in yields comparable to those obtained in earlier smaller scale trials (Scheme 5). Additionally, the amination reaction of compound 4a took place in the presence of La(OTf)3 using aliphatic amine and benzylamine as the amination reagents, giving compounds 6 and 7 in 79% and 84% yields, respectively. The hydrolysis of 4a to primary alcohol 8 was successfully carried out using LiAlH4 as the reductant, resulting in 91% yield.
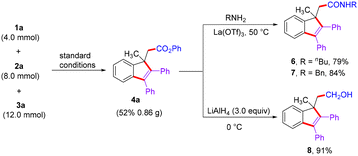 |
| Scheme 5 Gram-scale synthesis and transformations. | |
On the basis of our research results and previous reports,6,16 a proposed mechanism of this double carbopalladation/aryloxycarbonylation for the synthesis of indene-1-acetates is shown in Scheme 6. Initially, the oxidative addition of the Pd(0) species to the C–I bond of 1a forms intermediate A, and then intermolecular carbopalladation of the internal alkyne 2a occurs to afford B, which forms the alkylpalladium intermediate Cvia an intramolecular Heck carbopalladation. Then, the insertion of CO, released from phenyl formate 3a in the presence of K2CO3, gives the acyl Pd(II) species D. Finally, the reaction of intermediate D with phenol, which is generated from 3a, followed by reductive elimination delivers the desired product 4a and regenerates the active Pd(0) species.
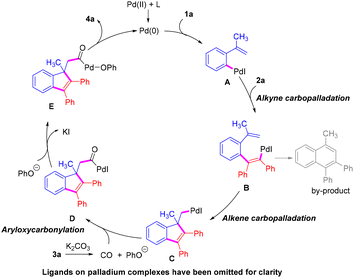 |
| Scheme 6 Plausible reaction mechanism. | |
Conclusions
In summary, we have developed a novel three-component cascade cyclization strategy to access all-carbon quaternary stereogenic center-possessing indene-1-acetates by a tandem double carbopalladation and aryloxycarbonylation under a palladium/air system. This protocol is compatible with a broad range of substrates and a wide range of functional groups and shows excellent selectivity. Moreover, the practical application of this protocol was showcased through the late-stage modification of bioactive molecules and gram-scale synthesis and transformations. Further research on the synthesis of functionalized indenes is presently underway.
Data availability
The authors declare that the data supporting the findings of this study are available within the paper and its ESI.† The experimental procedures, characterization data, and copies of NMR spectra are shown in the ESI.†
Conflicts of interest
The authors declare no competing financial interest.
Acknowledgements
This work was supported by grants from the National Natural Science Foundation of China (22201145), the Program of High-level Talents of Nantong University (03083031), the Science and Technology Project of Nantong City (JC12022052), and the Large Instruments Open Foundation of Nantong University.
References
-
(a) P. Hu, S. Lee, T. S. Herng, N. Aratani, T. P. Goncalves, Q. Qi, X. Shi, H. Yamada, K.-W. Huang, J. Ding, D. Kim and J. Wu, Toward Tetraradicaloid: The Effect of Fusion Mode on Radical Character and Chemical Reactivity, J. Am. Chem. Soc., 2016, 138, 1065–1077 CrossRef CAS;
(b) J. W. Huffman and L. W. Padgett, Recent Developments in the Medicinal Chemistry of Cannabimimetic Indoles, Pyrroles and Indenes, Curr. Med. Chem., 2005, 12, 1395–1411 CrossRef CAS;
(c) A. C. Grimsdale and K. Müllen, The Chemistry of Organic Nanomaterials, Angew. Chem., Int. Ed., 2005, 44, 5592–5629 CrossRef CAS;
(d) D. Chanda, D. Saikia, J. K. Kumar, J. P. Thakur, J. Agarwal, C. S. Chanotiya, K. Shanker and A. S. Negi, 1-Chloro-2-formyl indenes and tetralenes as antitubercular agents, Bioorg. Med. Chem. Lett., 2011, 21, 3966–3969 CrossRef CAS PubMed.
-
(a) X.-H. Duan, L.-N. Guo, H.-P. Bi, X.-Y. Liu and Y.-M. Liang, Highly Regioselective Synthesis of 2,3-Disubstituted Indenes via a Novel Palladium-Catalyzed Cyclization Reaction of Propargylic Carbonates with Carbon Nucleophiles, Org. Lett., 2006, 8, 5777–5780 CrossRef CAS;
(b) L.-N. Guo, X.-H. Duan, H.-P. Bi, X.-Y. Liu and Y.-M. Liang, Synthesis of Indenes via Palladium-Catalyzed Carboannulation of Diethyl 2-(2-(1-alkynyl)phenyl)malonate and Organic Halides, J. Org. Chem., 2006, 71, 3325–3327 CrossRef CAS;
(c) D. Zhang, Z. Liu, E. K. Yum and R. C. Larock, Synthesis of Indenes by the Transition Metal-Mediated Carboannulation of Alkynes, J. Org. Chem., 2007, 72, 251–262 CrossRef CAS PubMed.
-
(a) M. Egi, K. Shimizu, M. Kamiya, Y. Ota and S. Akai, Central-axial-central chirality transfer: asymmetric synthesis of highly substituted indenes bearing a stereogenic quaternary carbon center from optically active propargyl alcohols, Chem. Commun., 2015, 51, 380–383 RSC;
(b) D. Eom, S. Park, Y. Park, T. Ryu and P. H. Lee, Synthesis of Indenes via Brønsted Acid Catalyzed Cyclization of Diaryl- and Alkyl Aryl-1,3-dienes, Org. Lett., 2012, 14, 5392–5395 CrossRef CAS PubMed;
(c) X. Zhang, W. T. Teo, W. Rao, D.-L. Ma, C.-H. Leung and P. W. H. Chan, Synthesis of highly substituted indene derivatives by Brønsted acid catalyzed Friedel–Crafts reaction of homoallylic alcohols, Tetrahedron Lett., 2014, 55, 3881–3884 CrossRef CAS;
(d) D. H. Dethe and G. Murhade, Org. Lett., 2013, 15, 429–431 CrossRef CAS PubMed.
-
(a) L.-X. Shao, B. Xu, J.-W. Huang and M. Shi, Synthesis of the Indene, THF, and Pyrrolidine Skeletons by Lewis Acid Mediated Cycloaddition of Methylenecyclopropanes with Aldehydes, N-Tosyl Aldimines, and Acetals, Chem. – Eur. J., 2006, 12, 510–517 CrossRef;
(b) Y. Wang, P. R. McGonigal, B. Herlé, M. Besora and A. M. Echavarren, Gold(I) Carbenes by Retro-Buchner Reaction: Generation and Fate, J. Am. Chem. Soc., 2014, 136, 801–809 CrossRef CAS;
(c) S. Wang, Y. Zhu, Y. Wang and P. Lu, Synthesis of Functionalized Indenes via Cascade Reaction of Aziridines and Propargyl Alcohols, Org. Lett., 2009, 11, 2615–2618 CrossRef CAS.
-
(a) Y. Kuninobu, Y. Tokunaga, A. Kawata and K. Takai, Insertion of Polar and Nonpolar Unsaturated Molecules into Carbon−Rhenium Bonds Generated by C−H Bond Activation: Synthesis of Phthalimidine and Indene Derivatives, J. Am. Chem. Soc., 2006, 128, 202–209 CrossRef CAS;
(b) F. Zhou, M. Yang and X. Lu, Cationic Palladium(II)-Catalyzed Highly Enantioselective Tandem Reactions of ortho-Boronate-Substituted Cinnamic Ketones and Internal Alkynes: A Convenient Synthesis of Optically Active Indenes, Org. Lett., 2009, 11, 1405–1408 CrossRef CAS PubMed;
(c) J. Bucher, T. Stöβer, M. Rudolph, F. Rominger and A. S. K. Hashmi, CO Extrusion in Homogeneous Gold Catalysis: Reactivity of Gold Acyl Species Generated through Water Addition to Gold Vinylidenes, Angew. Chem., Int. Ed., 2015, 54, 1666–1670 CrossRef CAS;
(d) B. G. Das, A. Chirila, M. Tromp, J. N. H. Reek and B. Bruin, CoIII-Carbene Radical Approach to Substituted 1H-Indenes, J. Am. Chem. Soc., 2016, 138, 8968–8975 CrossRef CAS PubMed;
(e) S. Xu, R. Chen, Z. Fu, Q. Zhou, Y. Zhang and J. Wang, Palladium-Catalyzed Formal [4+1] Annulation via Metal Carbene Migratory Insertion and C(sp2)-H Bond Functionalization, ACS Catal., 2017, 7, 1993–1997 CrossRef CAS.
- For selected literature, see:
(a) H.-J. Tang, Y.-F. Zhang, Y.-W. Jiang and C. Feng, F– Nucleophilic-Addition-Induced [3+2] Annulation: Direct Access to CF3-Substituted Indenes, Org. Lett., 2018, 20, 5190–5193 CrossRef CAS PubMed;
(b) X. Abel-Snape, G. Wycich and M. Lautens, Synthesis of Indenes and Benzofulvenes via a Palladium-Catalyzed Three-Component Reaction, ACS Catal., 2022, 12, 3291–3301 CrossRef CAS;
(c) X. Jia, D. A. Petrone and M. Lautens, A Conjunctive Carboiodination: Indenes by a Double Carbopalladation-Reductive Elimination Domino Process, Angew. Chem., Int. Ed., 2012, 51, 9870–9872 CrossRef CAS;
(d) X.-W. Liu, S.-S. Li, D.-T. Dai, M. Zhao, C.-C. Shang, Y.-H. Xu and T.-P. Loh, Palladium-Catalyzed Dialkylation of C-C Triple Bonds: Access to Multi-Functionalized Indenes, Org. Lett., 2019, 21, 3696–3700 CrossRef CAS PubMed;
(e) T. Zhang, Y.-X. Luan, S.-J. Zheng, Q. Peng and M. Ye, Chiral Aluminum Complex Controls Enantioselective Nickel-Catalyzed Synthesis of Indenes: C−CN Bond Activation, Angew. Chem., Int. Ed., 2020, 59, 7439–7443 CrossRef CAS PubMed;
(f) C. Wang, C. Wu, Y. Yang, J. Xing and X. Dou, Rhodium-catalyzed formal [2+2+1] annulation of arylboronic acids with alkynes, Org. Chem. Front., 2022, 9, 6915–6919 RSC;
(g) S. Mondal, T. Ballav, T. S. Mohammad and V. Ganesh, Bis-benzofulvenes: Synthesis and Studies on Their Optoelectronic Properties, Org. Lett., 2023, 25, 3941–3945 CrossRef CAS;
(h) C. Han, F. Zhao, Q. Lu and F. Liu, Computational Determination of the Mechanism of the Palladium-Catalyzed Domino Reaction of ortho-Iodostyrene, Oxanorbornadiene, and Phenylboronic Acid, J. Org. Chem., 2023, 88, 15608–15614 CrossRef CAS PubMed.
-
(a) D. Zhang, E. K. Yum, Z. Liu and R. C. Larock, Synthesis of Indenes by the Palladium-Catalyzed Carboannulation of Internal Alkynes, Org. Lett., 2005, 7, 4963–4966 CrossRef CAS;
(b) X.-H. Duan, L.-N. Guo, H.-P. Bi, X.-Y. Liu and Y.-M. Liang, Synthesis of 2-Substitued 3-Aroylindenes via Palladium-Catalyzed Carbonylative Cyclization of Diethyl 2-(2-(1-Alkynyl)phenyl)malonates with Aryl Halides, Org. Lett., 2006, 8, 3053–3056 CrossRef CAS.
- For selected examples, see:
(a) L. Chen, C. Shi, W. Li, B. Li, J. Zhu, A. Lin and H. Yao, Palladium-Catalyzed Asymmetric C–C Bond Activation/Carbonylation of Cyclobutanones, Org. Lett., 2022, 24, 9157–9162 CrossRef CAS PubMed;
(b) Z. Yuan, Y. Zeng, Z. Feng, Z. Guan, A. Lin and H. Yao, Constructing chiral bicyclo[3.2.1]octanes via palladium-catalyzed asymmetric tandem Heck/carbonylation desymmetrization of cyclopentenes, Nat. Commun., 2020, 11, 2544 CrossRef CAS PubMed;
(c) K.-L. Song, B. Wu, W.-E. Gan, W.-C. Yang, X.-B. Chen, J. Cao and L.-W. Xu, Palladium-catalyzed gaseous CO-free carbonylative C–C bond activation of cyclobutanones, Org. Chem. Front., 2021, 8, 3398–3403 RSC;
(d) C. Chen, H. Zhao, Y. Pu, L. Tang, J. Wang and Y. Shang, Palladium-catalysed alkenyl and carbonylative C–C bond activation of cyclobutanones, Chem. Commun., 2021, 57, 12944–12947 RSC.
- For selected examples, see:
(a) H. Hu, F. Teng, J. Liu, W. Hu, S. Luo and Q. Zhu, Enantioselective Synthesis of 2-Oxindole Spirofused Lactones and Lactams by Heck/Carbonylative Cylization Sequences: Method Development and Applications, Angew. Chem., Int. Ed., 2019, 58, 9225–9229 CrossRef CAS PubMed;
(b) H. Hu, Y. Peng, T. Yu, S. Cheng, S. Luo and Q. Zhu, Palladium-Catalyzed Enantioselective 7-exo-Trig Carbopalladation/Carbonylation: Cascade Reactions To Achieve Atropisomeric Dibenzo[b,d]azepin-6-ones, Org. Lett., 2021, 23, 3636–3640 CrossRef CAS PubMed;
(c) C. Cheng, B. Wan, B. Zhou, Y. Gu and Y. Zhang, Enantioselective synthesis of quaternary 3,4-dihydroisoquinolinones via Heck carbonylation reactions: development and application to the synthesis of Minalrestat analogues, Chem. Sci., 2019, 10, 9853–9858 RSC;
(d) M. Chen, X. Wang, P. Yang, X. Kou, Z.-H. Ren and Z.-H. Guan, Palladium-Catalyzed Enantioselective Heck Carbonylation with a Monodentate Phosphoramidite Ligand: Asymmetric Synthesis of (+)-Physostigmine, (+)-Physovenine, and (+)-Folicanthine, Angew. Chem., Int. Ed., 2020, 59, 12199–12205 CrossRef CAS.
- L.-B. Jiang, X. Qi and X.-F. Wu, Benzene-1,3,5-triyl triformate (TFBen): a convenient, efficient, and non-reacting CO source in carbonylation reactions, Tetrahedron Lett., 2016, 57, 3368–3370 CrossRef CAS.
- P. Gautam, P. Kathe and B. M. Bhanage, Pd/C catalyzed phenoxycarbonylation using N-formylsaccharin as a CO surrogate in propylene carbonate, a sustainable solvent, Green Chem., 2017, 19, 823–830 RSC.
- M. Markovič, P. Lopatka, P. Koos and T. Gracza, Zn-Mediated Reduction of Oxalyl Chloride Forming CO and Its Application in Carbonylation Reactions, Org. Lett., 2015, 17, 5618–5621 CrossRef PubMed.
- For selected examples, see:
(a) J.-F. Carpentier, Y. Castanet, J. Brocard, A. Mortreux and F. Petit, A novel and convenient method for palladium-catalysed alkoxycarbonylation of aryl and vinyl halides using HCO2R/NaOR system, Tetrahedron Lett., 1991, 32, 4705–4708 CrossRef CAS;
(b) T. Ueda, H. Konishi and K. Manabe, Trichlorophenyl Formate: Highly Reactive and Easily Accessible Crystalline CO Surrogate for Palladium-Catalyzed Carbonylation of Aryl/Alkenyl Halides and Triflates, Org. Lett., 2012, 14, 5370–5373 CrossRef CAS PubMed;
(c) Z. Chen, L.-C. Wang and X.-F. Wu, Carbonylative synthesis of heterocycles involving diverse CO surrogates, Chem. Commun., 2020, 56, 6016–6030 RSC.
- For recent examples, see:
(a) J.-L. Liu, W. Wang, X. Qi and X.-F. Wu, Palladium-Catalyzed Reductive Aminocarbonylation of o-Iodophenol-Derived Allyl Ethers with o-Nitrobenzaldehydes to 3-Alkenylquinolin-2(1H)-ones, Org. Lett., 2022, 24, 2248–2252 CrossRef CAS;
(b) R.-R. Xu, D. Wen, X. Qi and X.-F. Wu, Palladium-catalyzed cascade Heck-type cyclization and reductive aminocarbonylation for the synthesis of functionalized amides, Org. Biomol. Chem., 2022, 20, 2605–2608 RSC;
(c) L. Yao, J. Ying and X.-F. Wu, Nickel-catalyzed cascade carbonylative synthesis of N-benzoyl indoles from 2-nitroalkynes and aryl iodides, Org. Chem. Front., 2021, 8, 6541–6545 RSC;
(d) Z.-P. Bao, R.-G. Miao, X. Qi and X.-F. Wu, A novel construction of acetamides from rhodium-catalyzed aminocarbonylation of DMC with nitro compounds, Chem. Commun., 2021, 57, 1955–1958 RSC.
- F. Sun, Y. Zheng, M. Wu, H. Ji, Z. Jiang, C. Liu and X.-X. Wu, Three-component cascade carbopalladation/Heck cyclization/borylation: facile access to boryl-functionalized indenes, Chem. Commun., 2024, 60, 8075–8078 RSC.
- H. Ye, L. Wu, M. Zhang, G. Jiang, H. Dai and X.-X. Wu, Palladium-catalyzed Heck cyclization/carbonylation with formates: synthesis of azaindoline-3-acetates and furoazaindolines, Chem. Commun., 2022, 58, 6825–6828 RSC.
- Product 4aj was recrystallized in dichloromethane, and the crystal structure of 4aj containing dichloromethane was confirmed by X-ray crystallographic analysis.
Footnotes |
† Electronic supplementary information (ESI) available. CCDC 2368290 (4aj). For ESI and crystallographic data in CIF or other electronic format see DOI: https://doi.org/10.1039/d4qo01570c |
‡ These authors contributed equally. |
|
This journal is © the Partner Organisations 2025 |
Click here to see how this site uses Cookies. View our privacy policy here.