DOI:
10.1039/D4QO01746C
(Research Article)
Org. Chem. Front., 2025,
12, 130-135
Synthesis of bambusurils with perfluoroalkylthiobenzyl groups as highly potent halide receptors†
Received
17th September 2024
, Accepted 25th October 2024
First published on 29th October 2024
Abstract
The preparation of anion receptors with ultrahigh binding affinities is an important, yet challenging, topic of supramolecular chemistry. The search for new structural motifs which would enhance the performance of anion receptors is therefore an important task. In this context, we report the synthesis of novel fluorinated bambus[6]urils that incorporate unique benzyl substituents with perfluoroalkylthio groups, aimed at enhancing their anion receptor capabilities. The synthetic strategy developed allows for the efficient preparation of these structural motifs. Ultrahigh stability of the complexes between halides and the prepared bambus[6]urils was observed and quantified using 19F NMR competition experiments. Replacing –CF3 groups on benzylated bambus[6]urils by –SCF3 groups increased the affinity of the macrocycles towards anions and provided the strongest iodide receptor reported with a binding affinity of 4 × 1013 M−1 in acetonitrile.
Introduction
Artificial host molecules with a high binding affinity towards anions find applications in detection systems for low anion concentrations, separation, environmental scavenging, or transmembrane transport.1–7 Macrocycles8–13 or cages14–17 are especially potent anion receptors as they encapsulate anions via multiple non-covalent interactions, often accompanied by complete anion desolvation. These host molecules are often valued not only for their high affinities but also for their high selectivity. However, their design and preparation are usually challenging. Structurally more simple acyclic host molecules are also capable of strong anion binding.18,19 This is generally achieved by the installation of electron-withdrawing substituents for higher polarization of the anion binding groups (such as urea or triazole). Phenyl moieties bearing –CF3, –NO2 or –F electron-withdrawing groups are commonly used in this context.20–22
The strongly electron-withdrawing –SCF3 group could be also attractive for this purpose, and it has a very high Hansch lipophilicity parameter as well.23,24 Several synthetic methods have been developed to incorporate the –SCF3 group into organic molecules, mostly to prepare new drug candidates.23,25–29 Surprisingly, no anion receptors which contain the –SCF3 group have been published in the literature to date other than the recently reported fluorinated bambus[6]urils (BUs).10,30,31 BUs are a class of deep cavity anion receptors, in which the anion binding is mediated via C–H hydrogen bonds, originating from sp3 hybridised carbon atoms. We have reported recently that the installation of –SCF3 groups to the para-position of their benzyl substituents dramatically increases the binding affinity of these macrocycles to anions, up to 1012 M−1 for BU5 and 1013 M−1 for BU7 in acetonitrile (Fig. 1).10,31
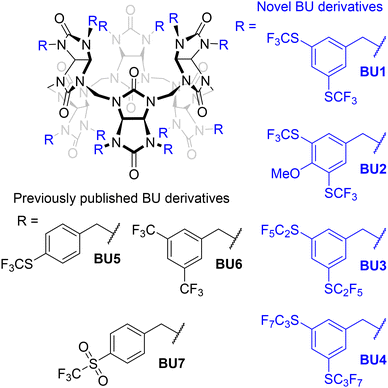 |
| Fig. 1 Structures of bambus[6]urils studied in this work. | |
This work presents the synthesis of BU derivatives bearing two electron-withdrawing –SCF3 or perfluoroalkylthio groups on their benzyl substituents (Fig. 1). The synthesis of 3,5-perfluoroalkylthiobenzyl moieties is reported for the first time. Furthermore, we have determined the association constants of the newly prepared macrocycles with chloride, bromide, and iodide using 19F NMR spectroscopy.
Results and discussion
Synthesis
For our study, we decided to synthesise BU1 with two –SCF3 groups per substituent as it can be directly compared with its previously studied analogue bearing two –CF3 groups. We also prepared BU2 bearing methoxy groups in addition to –SCF3 groups, allowing additional modifications of the macrocycle in the future. Macrocycles BU3 and BU4, with perfluoroethyl or perfluoropropyl groups attached to the sulphur atoms, were prepared to evaluate the influence of the length of the fluorinated alkyl chain on the properties of the macrocycle.
The synthesis started with the inexpensive 3,5-dihydroxybenzoic acid methyl ester 1 (for BU1, BU3 and BU4) or methoxy derivative 2
32 (for BU2) (Scheme 1a). The esters 1 and 2 were converted to the corresponding O-aryl dimethylcarbamothioates 3 and 4 by the reaction with dimethylthiocarbamoyl chloride (DMTCC) in the presence of 1,4-diazabicyclo[2.2.2]octane (DABCO) as a base. The intermediates 3
33 and 4 were obtained in high yields of 88% and 72%, respectively, on a multigram scale. To obtain S-aryl dimethylcarbamo-thioates 5 and 6, both 3 and 4 were converted by a Newman–Kwart rearrangement.33,34 The rearrangement of 3 to 5 was performed by heating a solution of 3 in diphenyl ether to reflux until a full conversion was observed, yielding 5 in a 94% yield.33 On the other hand, rearrangement of 4 to 6, was proven to be more challenging, particularly on a large scale, due to the presence of an electron-donating –OMe group. In contrast to 3, the conversion of 4 to 6 was slower and prolonging the reaction time increased the quantity of impurities resulting from the decomposition of either the product, starting material, or mono-rearranged compounds. Also, a high variation in the yield of 6 was obtained, ranging from 40 to 90%. Therefore, we tested several other reported conditions to perform the Newman–Kwart rearrangement, including heating of the reaction mixture by microwaves,35 using different solvents,36 or redox catalysis.37,38 Unfortunately, none of the alternative reaction conditions gave better results than the thermal rearrangement in diphenyl ether.
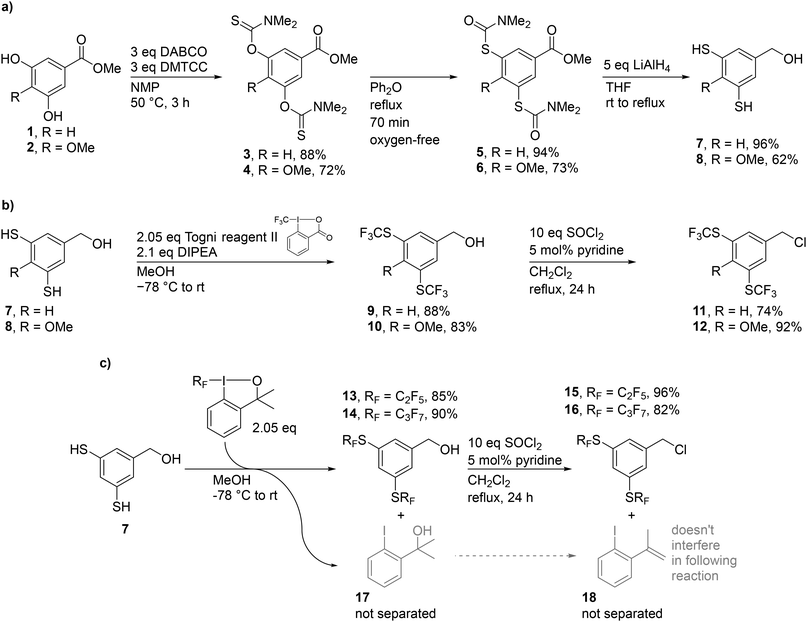 |
| Scheme 1 (a) Preparation of dithiol precursors 7 and 8 for all BUs; (b) preparation of fluorinated precursors 11 and 12 for BU1 and BU2; (c) preparation of precursors with perfluoroalkyl groups for BU3 and BU4. | |
The subsequent global reduction of 5 and 6 by an excess of lithium aluminium hydride provided dithiols 7
39 and 8 in 96% and 62% yields. The presence of the –OMe group in 8 resulted in a lower stability compared to 7. While dithiol 7 was obtained pure after the reduction, the more electron-rich dithiol 8, had to be purified by chromatography, was prone to decomposition by oxidation with atmospheric oxygen, and could not be stored for a prolonged time.
The dithiols 7 and 8 were trifluoromethylated using Togni reagent II. Either 7 or 8 was added into a degassed solution of Togni reagent II in MeOH in the presence of N,N-diisopropylethylamine (DIPEA) as a base. Compounds 9 and 10 were obtained after chromatographic separation in yields of 88% and 83%. The alcohols 9 and 10 were converted to the corresponding chlorides 11 and 12 by reaction with thionyl chloride.
To obtain precursors 13 and 14 with perfluoroalkylthio groups, we have used the commercially available Togni reagent I analogues bearing a perfluoroalkyl instead of –CF3 group (Scheme 1b). The obtained alcohols 13 and 14 with perfluoroalkylthio groups were converted to the corresponding chlorides 15 and 16. These synthetic steps were complicated by the Togni reagent byproduct 17 which was impossible to separate from 13 and 14 due to almost identical retention factors on normal-phase silica in various mobile-phase systems. Therefore, we have decided to use the mixture of 13 or 14 with 17 in the following reactions. Tertiary alcohol 17 underwent an elimination reaction forming alkene 18 by thionyl chloride during the conversion of alcohols 13 or 14 to chlorides 15 or 16. Compounds 15 and 16 were used in the following alkylation step as their mixtures with 18, for which separation was again not feasible.
Alkylation of p-methoxybenzyl-protected glycoluril 19 with the benzyl chlorides 11, 12, 15, and 16 provided tetrasubstituted glycolurils, which were used directly in the next step (Scheme 2).30,40 The p-methoxybenzyl groups were cleaved by ceric ammonium nitrate (CAN) and pure glycoluril building blocks 20–23 were obtained in yields ranging from 47% to 84%. The final step was the macrocyclization reaction of the corresponding glycoluril building blocks 20–23 with formaldehyde. The reactions were performed in 1,4-dioxane in the presence of sulphuric acid acting as an acid catalyst and as a source of HSO4− anions templating the formation of macrocycles BU1–BU4, with six glycoluril units, as their complexes with HSO4− in 54–74% yields.
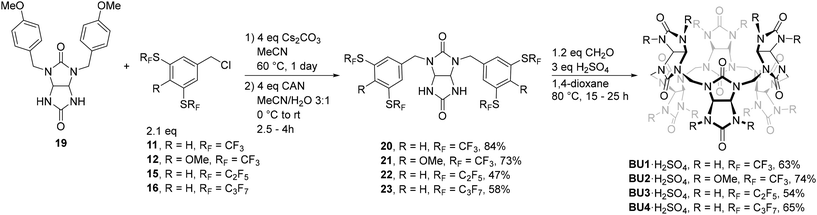 |
| Scheme 2 Preparation of BUs BU1–BU4. | |
Using Togni reagents with perfluorohexyl and perfluorooctyl groups to fluoroalkylate dithiol 7, we were able to prepare precursors with longer perfluoroalkyl chains. However, the drastic increase in the number of fluorine atoms in these molecules caused solubility issues during the preparation of corresponding glycoluril building blocks and ultimately prevented us from obtaining the other two highly fluorinated BUs (see the ESI† for the preparation of those glycolurils).
Anion binding studies
With the prepared BUs in hand, we decided to evaluate their affinity toward Cl−, Br−, and I−. This choice of anions is rationalised by the fact that I− is one of the strongest bound anions by BUs and the Cl− anion is one of the most investigated anions in binding by BUs,31,41,42 while it is also transported through lipid membranes by BUs.10,30 Association constants of the complexes were determined by competition experiments. In this method, we utilised previously prepared BU5 with known affinities to Cl−, Br−, and I−. Moreover, further competition experiments with BU6 or BU7 were conducted for further verification of the obtained Ka values (see the ESI, Section 4†). We prepared complexes of BU1–BU4 with Cl−, Br−, and I− and combined these with anion-free BU5 (or BU6) in acetonitrile. These competition experiments between pairs of BUs were monitored by 19F NMR spectroscopy.31 As the host–guest exchange process is slow on the NMR chemical shift time scale, signals corresponding to free and bound BUs appeared in the spectra and association constants could be calculated based on the integral intensities (Fig. 2).
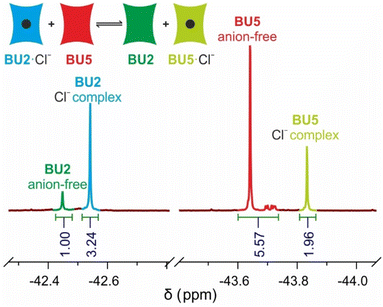 |
| Fig. 2
19F NMR spectrum of a competition experiment between BU2 and BU5 for Cl−, giving Ka(BU2)/Ka(BU5) = 9. | |
The obtained values are summarised in Table 1 together with the values for BU5–BU7 and allow comparison between the anion affinities of new and already reported BUs. BU1 with two –SCF3 groups per benzyl substituent binds chloride with log
Ka of 11.7, which is an order of magnitude stronger than BU5 having just a single –SCF3 group (log
Ka of 10.6). Similar trends were found for complexes of BU1 and BU5 with Br− and I−.
Table 1 Association constants (Ka) of BU – anion complexes
BU |
Substituent |
log Ka (Cl−) |
log Ka (Br−) |
log Ka (I−) |
meta
|
para
|
Determined by competition with BU5.
Taken from ref. 31.
Not determined.
|
BU1
|
SCF3 |
H |
11.7 ± 0.2a |
12.7 ± 0.2a |
13.6 ± 0.2a |
BU2
|
SCF3 |
OMe |
11.7 ± 0.2a |
12.8 ± 0.2a |
13.7 ± 0.2a |
BU5
|
H |
SCF3 |
10.6 ± 0.2b |
11.8 ± 0.2b |
12.2 ± 0.2b |
BU6
|
CF3 |
H |
11.2 ± 0.3a |
12.6 ± 0.2a |
—c |
BU7
|
H |
SO2CF3 |
11.5 ± 0.2b |
12.7 ± 0.2b |
13.1 ± 0.2b |
This result fits with the expectation that the introduction of more electron-withdrawing groups on BU substituents decreases the electron density inside the BU cavity. Rather surprising is the comparison of anion-binding affinities of BU6 and BU1. Changing –CF3 groups to –SCF3 increased the affinity of the BU macrocycle to Cl− about 3 times, which was confirmed by a direct competition study between these two compounds (Fig. S86†). In theory, the –CF3 and –SCF3 groups should have very a similar electron-withdrawing strength.24,43 However, there is no anion receptor series reported in which the effect of the insertion of a sulphur atom between the –CF3 group and the rest of the receptor on anion binding properties was investigated. Therefore, it is yet unclear whether this trend is more general, or specific for BU macrocycles.
The methoxy group as present in BU2 does not have any significant influence on the BU anion binding properties, as the competition experiments between BU1 and BU2 revealed essentially the same binding of these BUs to Cl− and I−. The methoxy group is a strongly electron-donating substituent by the mesomeric effect, but it weakly withdraws electrons from the aromatic ring by an inductive effect. Therefore, these two effects might cancel each other when considering the influence of the whole benzyl substituent on the electron density inside the BU cavity.
Competition between BU1 and BU5 for I− revealed that BU1 binds I− with log
Ka of 13.6, surpassing our previously reported strongest receptor BU7 (log
Ka of 13.1) for this anion,31 as well as other potent iodide receptors.44–46 Moreover, the direct competition experiment between BU2 and BU7 (Fig. S91†) confirmed that the affinity of BU2 to iodide is approximately 3 times higher than for BU7.
Unfortunately, for BU3 and BU4, the signals of anion-free and anion-complexed BU in 19F NMR spectra overlap, which made it impossible to quantify their binding to Cl−, Br−, and I−. However, we expect that these BUs would have either very similar or slightly higher binding than BU1 and BU2.
Conclusions
In this work, we have developed the synthesis of 3,5-perfluoroalkylthiobenzyl derivatives and used them for the preparation of four new bambusurils BU1–BU4, containing different bis(perfluoroalkylthio)benzyl substituents. The host–guest complexes of these new BUs with Cl−, Br−, and I− were investigated. We found that the binding affinity towards anions could be modulated by the type and number of the electron-withdrawing groups attached to the benzyl substituents of the bambusurils. These substituents made the BUs very effective anion receptors in MeCN. In particular, BU1 and BU2 and their complexes with iodide are characterised by a stability of log
Ka of 13.6 and 13.7, exceeding the previously published BU7 and making these novel macrocycles the strongest 1
:
1 receptors of iodide in any solvent ever reported. The methoxy-derivative BU2 is particularly attractive due to its good solubility in various solvents and its potential for conjugation with other species through the –OMe groups. In addition, the possibility of oxidation of the –SCF3 groups could increase the anion binding leading to even more potent receptors. The high anion affinities of the new BUs in combination with their lipophilic substituents suggest that these macrocycles hold promise as anion transporters. Investigations on this topic are ongoing in our laboratories. The reported synthetic approaches provide access to aromatic compounds bearing several –SCF3 groups and open opportunities for the preparation of other anion receptors containing these groups, which have been rarely reported in the literature.
Data availability
The data supporting this article have been included as part of the ESI.†
Conflicts of interest
There are no conflicts to declare.
Acknowledgements
This work was supported by the Czech Science Foundation (23-05271S). We thank the RECETOX Research Infrastructure (LM2023069) financed by the Ministry of Education, Youth and Sports. This project was supported from the European Union's Horizon 2020 Research and Innovation Programme under grant agreement no. 857560 (CETOCOEN Excellence). This publication reflects only the author's view and the European Commission is not responsible for any use that may be made of the information it contains. MC is a Research Fellow and HV is a Research Associate of the Fonds de la Recherche Scientifique—FNRS. We acknowledge Proteomic Core Facility of CIISB, Instruct-CZ Centre, supported by MEYS CR (LM2018127) and the National Infrastructure for Chemical Biology (CZ-OPENSCREEN, LM2023052). This article is also based upon work from COST Action CA22131, supported by COST (European Cooperation in Science and Technology).
References
- B. A. McNally, A. V. Koulov, T. N. Lambert, B. D. Smith, J.-B. Joos, A. L. Sisson, J. P. Clare, V. Sgarlata, L. W. Judd, G. Magro and A. P. Davis, Structure–Activity Relationships in Cholapod Anion Carriers: Enhanced Transmembrane Chloride Transport through Substituent Tuning, Chem. – Eur. J., 2008, 14, 9599–9606 CrossRef CAS
.
- H. Zhou, Y. Zhao, G. Gao, S. Li, J. Lan and J. You, Highly Selective Fluorescent Recognition of Sulfate in Water by Two Rigid Tetrakisimidazolium Macrocycles with Peripheral Chains, J. Am. Chem. Soc., 2013, 135, 14908–14911 CrossRef CAS PubMed
.
- D. Banerjee, D. Kim, M. J. Schweiger, A. A. Kruger and P. K. Thallapally, Removal of TcO4− ions from solution: materials and future outlook, Chem. Soc. Rev., 2016, 45, 2724–2739 RSC
.
- L. Qin, S. J. N. Vervuurt, R. B. P. Elmes, S. N. Berry, N. Proschogo and K. A. Jolliffe, Extraction and transport of sulfate using macrocyclic squaramide receptors, Chem. Sci., 2020, 11, 201–207 RSC
.
- E. J. Mitchell, A. J. Beecroft, J. Martin, S. Thompson, I. Marques, V. Félix and P. D. Beer, Hydrosulfide (HS−) Recognition and Sensing in Water by Halogen Bonding Hosts, Angew. Chem., Int. Ed., 2021, 60, 24048–24053 CrossRef CAS PubMed
.
- L. K. Macreadie, A. M. Gilchrist, D. A. McNaughton, W. G. Ryder, M. Fares and P. A. Gale, Progress in anion receptor chemistry, Chem, 2022, 8, 46–118 CAS
.
- A. Gogoi, D. Dutta, B. Gil-Hernández and S. K. Dey, Anion-exchange facilitated selective extraction of sulfate and phosphate by overcoming the Hofmeister bias, RSC Adv., 2023, 13, 16185–16195 RSC
.
- E. A. Katayev, N. V. Boev, E. Myshkovskaya, V. N. Khrustalev and Yu. A. Ustynyuk, Expanding Sapphyrin: Towards Selective Phosphate Binding, Chem. – Eur. J., 2008, 14, 9065–9073 CrossRef CAS
.
- Y. Haketa and H. Maeda, From Helix to Macrocycle: Anion-Driven Conformation Control of π-Conjugated Acyclic Oligopyrroles, Chem. – Eur. J., 2011, 17, 1485–1492 CrossRef CAS
.
- H. Valkenier, O. Akrawi, P. Jurček, K. Sleziaková, T. Lízal, K. Bartik and V. Šindelář, Fluorinated Bambusurils as Highly Effective and Selective Transmembrane Cl−/HCO3− Antiporters, Chem, 2019, 5, 429–444 Search PubMed
.
- T. Bunchuay, K. Boonpalit, A. Docker, A. Ruengsuk, J. Tantirungrotechai, M. Sukwattanasinitt, P. Surawatanawong and P. D. Beer, Charge neutral halogen bonding tetradentate-iodotriazole macrocycles capable of anion recognition and sensing in highly competitive aqueous media, Chem. Commun., 2021, 57, 11976–11979 RSC
.
- X. Wu, P. Wang, W. Lewis, Y.-B. Jiang and P. A. Gale, Measuring anion binding at biomembrane interfaces, Nat. Commun., 2022, 13, 4623 CrossRef CAS PubMed
.
- S. Sarkar, P. Ballester, M. Spektor and E. A. Kataev, Micromolar Affinity and Higher: Synthetic Host–Guest Complexes with High Stabilities, Angew. Chem., Int. Ed., 2023, 62, e202214705 CrossRef CAS PubMed
.
- Y. Liu, W. Zhao, C.-H. Chen and A. H. Flood, Chloride capture using a C–H hydrogen-bonding cage, Science, 2019, 365, 159 CrossRef CAS PubMed
.
- J. Bartl and S. Kubik, Anion Binding of a Cyclopeptide-Derived Molecular Cage in Aqueous Solvent Mixtures, ChemPlusChem, 2020, 85, 963–969 CrossRef CAS PubMed
.
- C. Mao, R. Wu, N. Chen, H. Zheng, Y. Cai, L. Kong and X. Hu, Synthesis and anion
recognition characteristics of a trapezoidal benzene cage, Org. Chem. Front., 2024, 11, 3348–3357 RSC
.
- L. Jing, E. Deplazes, J. K. Clegg and X. Wu, A charge-neutral organic cage selectively binds strongly hydrated sulfate anions in water, Nat. Chem., 2024, 16, 335–342 CrossRef CAS PubMed
.
- N. Busschaert, S. J. Bradberry, M. Wenzel, C. J. E. Haynes, J. R. Hiscock, I. L. Kirby, L. E. Karagiannidis, S. J. Moore, N. J. Wells, J. Herniman, G. J. Langley, P. N. Horton, M. E. Light, I. Marques, P. J. Costa, V. Félix, J. G. Frey and P. A. Gale, Towards predictable transmembrane transport: QSAR analysis of anion binding and transport, Chem. Sci., 2013, 4, 3036–3045 RSC
.
- L. E. Bickerton, A. J. Sterling, P. D. Beer, F. Duarte and M. J. Langton, Transmembrane anion transport mediated by halogen bonding and hydrogen bonding triazole anionophores, Chem. Sci., 2020, 11, 4722–4729 RSC
.
- J. P. Clare, A. J. Ayling, J.-B. Joos, A. L. Sisson, G. Magro, M. N. Pérez-Payán, T. N. Lambert, R. Shukla, B. D. Smith and A. P. Davis, Substrate Discrimination by Cholapod Anion Receptors: Geometric Effects and the “Affinity–Selectivity Principle”, J. Am. Chem. Soc., 2005, 127, 10739–10746 CrossRef CAS PubMed
.
- H. Li, H. Valkenier, L. W. Judd, P. R. Brotherhood, S. Hussain, J. A. Cooper, O. Jurček, H. A. Sparkes, D. N. Sheppard and A. P. Davis, Efficient, non-toxic anion transport by synthetic carriers in cells and epithelia, Nat. Chem., 2016, 8, 24–32 CrossRef CAS PubMed
.
- A. Docker, C. H. Guthrie, H. Kuhn and P. D. Beer, Modulating Chalcogen Bonding and Halogen Bonding Sigma-Hole Donor Atom Potency and Selectivity for Halide Anion Recognition, Angew. Chem., Int. Ed., 2021, 60, 21973–21978 CrossRef CAS PubMed
.
- X.-H. Xu, K. Matsuzaki and N. Shibata, Synthetic Methods for Compounds Having CF3–S Units on Carbon by Trifluoromethylation, Trifluoromethylthiolation, Triflylation, and Related Reactions, Chem. Rev., 2015, 115, 731–764 CrossRef CAS
.
- C. Hansch, A. Leo and R. W. Taft, A survey of Hammett substituent constants and resonance and field parameters, Chem. Rev., 1991, 91, 165–195 CrossRef CAS
.
- M. Hamzehloo, A. Hosseinian, S. Ebrahimiasl, A. Monfared and E. Vessally, Direct C-H trifluoromethylthiolation of (hetero)arenes: A review, J. Fluor. Chem., 2019, 224, 52–60 CrossRef CAS
.
- V. N. Boiko, Aromatic and heterocyclic perfluoroalkyl sulfides. Methods of preparation, Beilstein J. Org. Chem., 2010, 6, 880–921 CrossRef
.
- Y.-M. Lin, L. Q. Jiang and W.-B. Yi, Trifluoromethanesulfonyl-Based Reagents for Direct Trifluoromethylthiolation through Deoxygenative Reduction, Asian J. Org. Chem., 2019, 8, 627–636 CrossRef CAS
.
- Y. Yang, S. Miraghaee, R. Pace, T. Umemoto and G. B. Hammond, Preparation and Reactivity Study of a Versatile Trifluoromethylthiolating Agent: S-Trifluoromethyl Trifluoromethanesulfonothioate (TTST), Angew. Chem., Int. Ed., 2023, 62, e202306095 CrossRef CAS
.
- L. J. N. Waddell, C. Wilson and A. Sutherland, Trifluoromethylthiolation of Arenes Using Lewis Acid and Lewis Base Dual Catalysis, J. Org. Chem., 2024, 89, 1275–1284 CrossRef CAS PubMed
.
- N. A. De Simone, M. Chvojka, J. Lapešová, L. Martínez-Crespo, P. Slávik, J. Sokolov, S. J. Butler, H. Valkenier and V. Šindelář, Monofunctionalized Fluorinated Bambusurils and Their Conjugates for Anion Transport and Extraction, J. Org. Chem., 2022, 87, 9829–9838 CrossRef CAS
.
- M. Chvojka, D. Madea, H. Valkenier and V. Šindelář, Tuning CH Hydrogen Bond-Based Receptors toward Picomolar Anion Affinity via the Inductive Effect of Distant Substituents, Angew. Chem., Int. Ed., 2024, 63, e202318261 CrossRef CAS PubMed
.
- J. Zhu, J. Chastanet and R. Beugelmans, Selective Hydroxy Group Protection of Gallic Acid, Synth. Commun., 1996, 26, 2479–2486 CrossRef CAS
.
- P. T. Corbett, J. K. M. Sanders and S. Otto, Exploring the Relation between Amplification and Binding in Dynamic Combinatorial Libraries of Macrocyclic Synthetic Receptors in Water, Chem. – Eur. J., 2008, 14, 2153–2166 CrossRef CAS PubMed
.
- G. C. Lloyd-Jones, J. D. Moseley and J. S. Renny, Mechanism and Application of the Newman-Kwart O→S Rearrangement of O-Aryl Thiocarbamates, Synthesis, 2008, 661–689 CAS
.
- J. D. Moseley and P. Lenden, A high temperature investigation using microwave synthesis for electronically and sterically disfavoured substrates of the Newman–Kwart rearrangement, Tetrahedron, 2007, 63, 4120–4125 CrossRef CAS
.
- J. D. Moseley, R. F. Sankey, O. N. Tang and J. P. Gilday, The Newman–Kwart rearrangement re-evaluated by microwave synthesis, Tetrahedron, 2006, 62, 4685–4689 CrossRef CAS
.
- S. K. Pedersen, A. Ulfkjær, M. N. Newman, S. Yogarasa, A. U. Petersen, T. I. Sølling and M. Pittelkow, Inverting the Selectivity of the Newman–Kwart Rearrangement via One Electron Oxidation at Room Temperature, J. Org. Chem., 2018, 83, 12000–12006 CrossRef CAS PubMed
.
- T. Gendron, R. Pereira, H. Y. Abdi, T. H. Witney and E. Årstad, Iron(II)/Persulfate Mediated Newman–Kwart Rearrangement, Org. Lett., 2020, 22, 274–278 CrossRef CAS PubMed
.
-
H. Miki, T. Nakahama, S. Yokoyama and S. Mashiko, US Pat., US6630578B2, 2003 Search PubMed
.
- V. Havel, T. Sadilová and V. Šindelář, Unsubstituted Bambusurils: Post-Macrocyclization Modification of Versatile Intermediates, ACS Omega, 2018, 3, 4657–4663 CrossRef CAS
.
- V. Havel and V. Sindelar, Anion Binding Inside a Bambus[6]uril Macrocycle in Chloroform, ChemPlusChem, 2015, 80, 1601–1606 CrossRef CAS PubMed
.
- T. Fiala, K. Sleziakova, K. Marsalek, K. Salvadori and V. Sindelar, Thermodynamics of Halide Binding to a Neutral Bambusuril in Water and Organic Solvents, J. Org. Chem., 2018, 83, 1903–1912 CrossRef CAS
.
- D. Shin and Y. Jung, Molecular electrostatic potential as a general and versatile indicator for electronic substituent effects: statistical analysis and applications, Phys. Chem. Chem. Phys., 2022, 24, 25740–25752 RSC
.
- S. Lee, C.-H. Chen and A. H. Flood, A pentagonal cyanostar macrocycle with cyanostilbene CH donors binds anions and forms dialkylphosphate [3]rotaxanes, Nat. Chem., 2013, 5, 704–710 CrossRef CAS PubMed
.
- A. Borissov, I. Marques, J. Y. C. Lim, V. Félix, M. D. Smith and P. D. Beer, Anion Recognition in Water by Charge-Neutral Halogen and Chalcogen Bonding Foldamer Receptors, J. Am. Chem. Soc., 2019, 141, 4119–4129 CrossRef CAS PubMed
.
- Y. Wu, C. Zhang, S. Fang, D. Zhu, Y. Chen, C. Ge, H. Tang and H. Li, A Self-Assembled Cage Binding Iodide Anions over Other Halide Ions in Water, Angew. Chem., Int. Ed., 2022, 61, e202209078 CrossRef CAS PubMed
.
Footnote |
† Electronic supplementary information (ESI) available: Synthetic procedures, characterization of new compounds and binding studies. See DOI: https://doi.org/10.1039/d4qo01746c |
|
This journal is © the Partner Organisations 2025 |
Click here to see how this site uses Cookies. View our privacy policy here.