DOI:
10.1039/D4RA09098E
(Paper)
RSC Adv., 2025,
15, 2066-2077
Structural and luminescent properties of a Cr3+/Sm3+ doped GdAlO3 orthorhombic perovskite for solid-state lighting applications
Received
30th December 2024
, Accepted 11th January 2025
First published on 23rd January 2025
Abstract
The Cr3+ and Sm3+ doped GdAlO3 perovskite with formula Gd0.995Sm0.005Al0.995Cr0.005O3, was synthesized via a solid-state reaction method, and its structure, morphology, and photoluminescence properties were thoroughly investigated. The compound crystallizes in the orthorhombic Pbnm space group, with Cr3+ transition-metal ions substituting Al3+ in the octahedral symmetry site, and Sm3+ lanthanide (rare-earth) ions occupying the tetrahedral site. The material's morphology and chemical composition homogeneity were evaluated through Scanning Electron Microscopy (SEM) and Energy Dispersive X-ray analysis. Photoluminescence excitation (PLE) and emission spectra (PL) were used to shed light on the electronic structure of the Cr3+ cations, through crystal field analysis in the Oh symmetry site. Theoretical studies enabled the precise assignment of the Cr3+ 3d–3d transitions. The intra-configurational 4f–4f transitions of Sm3+ resulted in a variety of excitation bands appearing in the high-wavelength range of the PLE spectrum. The photoluminescence studies supported the occurrence of energy transfer in the doped GdAlO3 perovskite between Gd3+, Sm3+ and Cr3+ ions. The obtained results suggest the high potential of the synthesized material for solid state lighting applications.
1. Introduction
The investigation of rare-earth-doped luminescent materials has unlocked new avenues of innovation, driving advancements across various high-tech industries.1 Among these, white light-emitting devices (LEDs) stand out due to their exceptional luminescent properties. In the context of global energy challenges and the growing threat of climate change, the need for innovative and efficient phosphors tailored for solid-state lighting (SSL) is critical.2,3 The demand for novel phosphors used in SSL displays and lamps continues to rise, driven by their prolonged lifespan, high luminescence efficiency, and contribution to sustainable environmental practices.4–8
Aluminate-based host matrices have garnered significant attention as ideal candidates for phosphor development due to their superior crystallinity, excellent luminous characteristics, straightforward synthesis, favorable color parameters, and exceptional chemical and thermal stability. Their high heat resistance further enhances their suitability for doping with various ions, enabling the creation of materials optimized for diverse applications.9,10 Consequently, aluminate-based materials have been extensively investigated and have found applications in energy harvesting for flat-panel displays,11 SSL,12 scintillators,13 optical lasers,14 light-emitting diodes,15 photonic storage devices, and remote sensing technologies.16
Among these materials, gadolinium aluminate (GdAlO3), featuring an orthorhombic perovskite crystal structure (Pnma space group), is a particularly notable example. GdAlO3 is uniquely suited for multifunctional applications due to its robust thermal and chemical stability, compatibility with rare-earth dopants, and excellent energy transfer characteristics. These properties make it an attractive host matrix for developing phosphors with tailored optical and magnetic functionalities. While gadolinium can be expensive, the strategic use of GdAlO3 in doped compositions mitigates costs,17 and its performance advantages justify its use in niche applications such as magneto-optoelectronics and hybrid devices requiring simultaneous photoluminescent and magnetic properties.18–20 Furthermore, GdAlO3 based materials show promise for energy storage and conversion technologies, adding to their appeal as next-generation phosphor materials.
Cr3+ ions were selected as primary activators due to their well-established photoluminescent properties, including intense and tunable red emissions. To further enhance the material's spectral characteristics, Sm3+ was introduced as a codopant. Sm3+ was chosen for its ability to enhance red luminescence and its potential to act as an energy transfer mediator, facilitating a synergistic effect with Cr3+. This combination is particularly relevant for applications requiring optimized spectral output, such as full-spectrum lighting or display technologies. Moreover, the dopant combination leverages the perovskite structure of GdAlO3 to enable efficient energy transfer and tailored luminescent properties.
In this investigation, we synthesized and characterized the structural and luminescence properties of a Cr3+ doped orthorhombic GdAlO3 perovskite phosphor, codoped with Sm3+ to achieve the composition Gd0.995Sm0.005Al0.995Cr0.005O3. This study aims to elucidate the fundamental mechanisms governing the optical properties of GdAlO3 doped with Cr3+ and Sm3+, thereby fostering further exploration into the potential of complex oxides doped with transition and rare-earth elements for advanced technological applications. The findings presented here demonstrate the promise of this novel material for applications in solid-state lighting.
2. Materials and methods
In this study, GdAlO3 perovskite was synthesized via solid-state method. High-purity gadolinium oxide (Gd2O3) and aluminum oxide (Al2O3) powders were weighted in stoichiometric ratio to form the base material. To introduce chromium and samarium doping, appropriate amounts of samarium oxide (Sm2O3) and chromium oxide (Cr2O3) were added, ensuring the desired doping level of Gd0.995Sm0.005Al0.995Cr0.005O3. The solid-state method was chosen for its robustness and ability to exert precise control over reaction conditions. Following pre-annealing at 700 °C for 24 hours to remove volatile inorganic materials, the mixture was ground using an agate mortar and pestle to ensure intimate mixing of the reactants. The resulting mixture was then sintered at 1200 °C for 24 hours, promoting atomic and ionic diffusion essential for the formation of the desired doped GdAlO3 compound. The high temperature during sintering facilitated the incorporation of Cr3+ and Sm3+ ions into the crystal lattice, ensuring the development of the desired orthorhombic crystal structure. Finally, the sintered material was compacted into pellets with a diameter of 8 mm, which were used in the subsequent characterization and analyses experiments.
Characterization of the newly synthesized compound was undertaken using different techniques. The material was first analyzed by powder X-ray diffraction (X-ray Siemens D5000, with λ = 1.5406 Å CuKα radiation). Data was collected within the 20–100° 2θ range, with a step size of 0.02°. Subsequently, photoluminescence emission (PL) and excitation (PLE) measurements were collected in a Horiba-Jobin-Yvon Fluorolog 322, operating in time-resolved mode (using a pulsed lamp) with a 0.05 ms delay after-flash. The morphology of the material and its chemical homogeneity were investigated by scanning electron microscopy (SEM), using a TESCAN VEGA3 SBH instrument equipped with an X-ray energy dispersive microscopy (EDS) detector. Image analysis was carried out using the ImageJ software,21 and structure analysis was undertaken using the Visualization Electronic and Structural Analysis (VESTA) software.22–24
Raman spectra were collected (with 4 cm−1 spectral resolution) in a LabRAM HR Evolution Raman microscope (Horiba Scientific) with a 600 g mm−1 grating. The excitation was provided by a He–Ne laser (λ = 633 nm), which was focused on the sample to a ∼1 mm spot using a 100× objective. The applied laser power of ∼0.2 mW at the sample resulted in no detectable heating or degradation. The collection time was 10 s with 100 accumulations being averaged to generate the final spectra.
The chromaticity coordinates (x, y) of the synthesized Gd0.995Sm0.005Al0.995Cr0.005O3 phosphor were determined using the CIE 1931 color space from its emission spectrum under 377 nm excitation. The correlated color temperature (CCT) and color purity (CP) values were calculated based on established methods.25,26 The resulting parameters are presented in Table 1, demonstrating the material's potential for applications in warm white light-emitting diodes (LEDs).
Table 1 CIE parameters for the red luminescence color of the synthesized Cr3+/Sm3+ doped GdAlO3 phosphor
x |
y |
CCT (K) |
CP (%) |
0.62157 |
0.37383 |
1892.46 |
87.08 |
Fig. 1 shows the CIE 1931 chromaticity diagram for the Gd0.995Sm0.005Al0.995Cr0.005O3 phosphor, generated from the emission spectrum under 377 nm excitation, where the coordinates are positioned in the reddish-orange region.
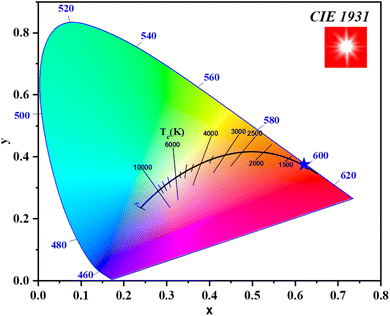 |
| Fig. 1 The CIE graph for the studied Cr3+/Sm3+ doped GdAlO3 phosphor. | |
3. Results and discussion
3.1 Powder X-ray diffraction analyses
Powder X-ray diffraction (PXRD) is a powerful technique for elucidating crystal phases, lattice parameters, and overall structural characteristics of solid materials. To determine the phases present in the synthesized material, its PXRD diffraction pattern (Fig. 2) was obtained and compared with ICDD (International Center for Diffraction Data) database. The observed peaks could be successfully indexed to a primitive orthorhombic crystal lattice, within the Pbnm space group. The identification process used standard JCPDS data files [GdAlO3 46-0395; Gd2O3 88-2165]. Rietveld refinement revealed the presence of two phases, the predominant one corresponding to the perovskite compound and the minor to Gd2O3. The presence of the Gd2O3 as impurity in the sample is consistent with previous studies,27 where this impurity was also observed. Table 2 presents the refined crystallographic parameters, with the agreement between calculated and observed profiles being satisfactory, as indicated by a χ2 value of 1.7. The fit quality between the observed and calculated diffraction patterns is reflected by Rp = 17.6, Rwp = 14.2, and Re = 10.9. While, Rp (profile R-factor) is a measure of the agreement between the observed and calculated diffraction profiles, Rwp (weighted profile R-factor) is similar to Rp but takes into account the statistical weights of the observed intensities and Re (expected R-factor) represents the ideal R-factor that would be obtained if the model perfectly fits the data, considering only statistical uncertainties. Fig. 3 depicts the structures of the GdAlO3 perovskite, where Al3+ and Gd3+ cations are bonded to six and eight oxygen atoms, respectively. Al3+ ions occupy an octahedral (Oh) coordination site corresponding to corner-sharing AlO6 octahedra with tilt angles of 24°, while Gd3+ stay in a coordination site whose symmetry is Cs. The compound presents four shorter and two longer Al–O bond lengths, along with two inequivalent O2− sites. In the first site, O2− is bonded in a 5-coordination geometry to three equivalent Gd3+ and two equivalent Al3+ cations; in the second, O2− is bonded in a 4-coordination geometry to two equivalent Gd3+ and two equivalent Al3+ cations. Upon doping, Cr3+ and Sm3+ cations are hypothesized to replace Al3+ and Gd3+, respectively, due to the close match in their ionic radii. Cr3+ (0.615 Å in octahedral coordination) is similar in size to Al3+ (0.535 Å), making it a suitable substitute at the Al3+ site. Similarly, the ionic radius of Sm3+ (1.079 Å in nine-fold coordination) closely matches that of Gd3+ (1.053 Å), facilitating substitution at the Gd3+ site.
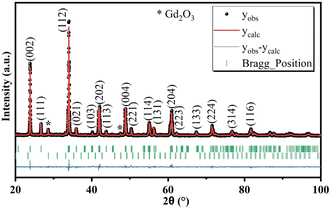 |
| Fig. 2 Results of the performed Rietveld refinement for Gd0.995Sm0.005Al0.995Cr0.005O3. | |
Table 2 Cell parameters and volume resulting from the Rietveld refinement for the synthesized compound, and statistical indicators
Formula |
Gd0.995Sm0.005Al0.995Cr0.005O3 |
Rp |
17.6 |
Space group |
Pbnm |
Rwp |
14.2 |
a (Å) |
5.2517 |
Re |
10.9 |
b (Å) |
5.2998 |
χ2 |
1.7 |
c (Å) |
7.4458 |
Gd2O3 (%) |
3.0501 |
V (Å3) |
207.2401 |
SEM (nm) |
177 |
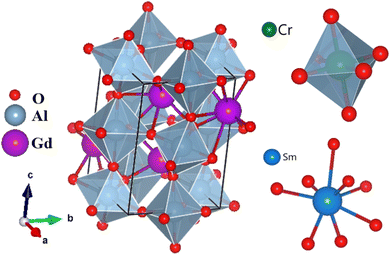 |
| Fig. 3 Illustration (Vesta software images) of the structure of the GdAlO3 perovskite, and of the 8-coordinated SmO8 and 6-coordinated (octahedral) CrO6 units. | |
3.2 EDS and particles morphology studies
Scanning electron microscopy (SEM) was employed to investigate the morphology of particles of the synthesized compound. Fig. 4 shows the presence of agglomerated and irregularly shaped grains, resulting from the substantial surface area of the particles and their interaction via weak van der Waals forces. The average particle size distribution, determined by Lorentz fitting using the ImageJ software, shows a peak centered at 177 nm.
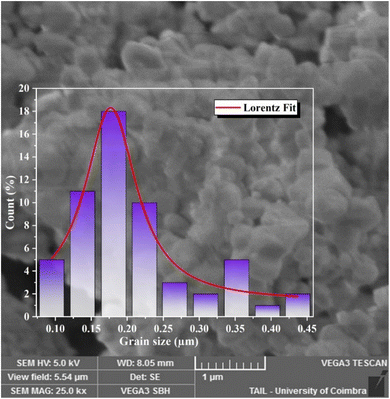 |
| Fig. 4 SEM image and particle size distribution histogram of the synthesized compound, and results of the Lorentz fit to the distribution data. | |
To confirm the chemical composition of the sample, its EDS spectrum was collected (Fig. 5). The spectrum exhibits characteristic peaks of all expected elements (Gd, Cr, Al, Sm, O) confirming the presence of these elements in the sample. The low-energy peaks correspond to lighter elements like Al and O, while the intermediate-energy peaks are attributed to Cr, Sm, and Gd, with distinct signals from their L and K edges. High-energy peaks, primarily from the K edges of Gd, also show some overlap from Sm, consistent with their atomic characteristics. All peaks are clearly labeled in the spectrum for clarity.
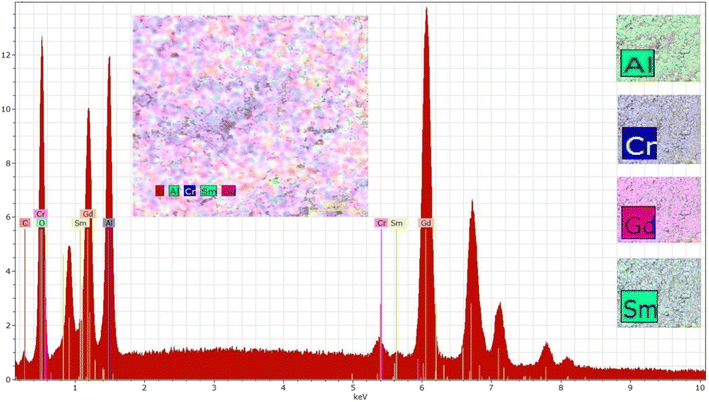 |
| Fig. 5 EDS spectrum of Gd0.995Sm0.005Al0.995Cr0.005O3 and elements distribution maps. | |
The elemental distribution maps, presented in the smaller images on the right of Fig. 5, provide a visual representation of the spatial distribution of the elements in the sample. While the main inset (colorful grain map) shows some inhomogeneity in the intensity of elemental signals, this variation is likely due to factors such as sample morphology, local concentration differences, or surface roughness, which can affect signal intensities. Despite these minor variations, the maps demonstrate significant co-localization of the elements, indicating a uniform distribution across the sample. This is further supported by the composite overlay of the elemental maps, where no regions are completely absent of any detected elements, confirming the overall homogeneity of the elemental distribution.
Table 3 provides the results of the quantitative analysis of the elements present in the sample, which show good agreement with the theoretical values for the expected composition. The observed differences between the nominal composition and EDS results, particularly for Cr and Sm, are attributed to the limitations of EDS in quantifying trace elements and matrix effects. Despite these minor discrepancies, the elemental maps confirm a homogeneous distribution of elements across the sample.
Table 3 Elemental composition obtained from the EDS data of the studied sample and theoretical values for Gd0.995Sm0.005Al0.995Cr0.005O3
Element |
Series |
Experimental (weight%) |
Error (%) |
Theoretical (weight%) |
Experimental (atomic%) |
Al |
K |
16.19 |
1.61 |
14.57 |
17.56 |
Cr |
K |
0.30 |
0.09 |
0.14 |
0.23 |
Sm |
L |
2.92 |
0.18 |
0.41 |
1.13 |
Gd |
L |
80.59 |
3.92 |
84.88 |
81.08 |
Total |
100.00 |
|
100.00 |
100.00 |
3.3 Raman spectroscopy analyses
The symmetry analyses of Pbnm space group symmetry perovskites of general formula ABO3 has been detailed by Chopelas, who has performed a systematic study of the Raman spectra of 30 different aluminate orthorhombic perovskites with emphasis on YAlO3 and GdAlO3.28 In the Brillouin zone center, the 24 Raman active optical modes span the symmetry species 7Ag + 7B1g + 5B2g + 5B3g. Chopelas has observed and assigned twenty of these modes for GdAlO3 using single crystal polarized Raman spectroscopy with excitation at 488.0 or 457.9 nm (Ar+ laser).28
The Raman spectra of GdAlO3 and of the synthesized Gd0.995Sm0.005Al0.995Cr0.005O3 compound obtained in the present study using 633 nm excitation are shown in Fig. 6, together with the difference spectrum obtained by subtracting the spectrum of the undoped GdAlO3 perovskite from that of the newly prepared compound. The difference spectrum allows to better notice the spectral changes due to the presence of the dopant atoms, which for most of the bands correspond to small shifts to lower frequency. These changes could be anticipated considering the much larger mass of chromium compared to aluminum (51.996 vs. 26.9815). On the other hand, the mass difference between gadolinium and samarium is small (157.2 vs. 150.4) so that the effects of the substitution of Gd3+ ions by Sm3+ at the level of substitution in the studied compound could be expected to lead to experimentally non-detectable perturbations in the frequencies of the modes where the movements of these atoms play a major role.
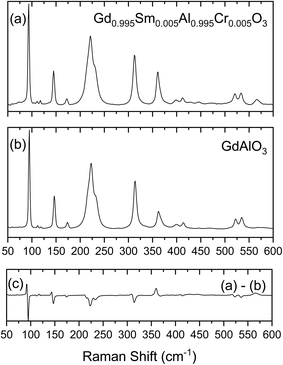 |
| Fig. 6 Raman spectra of the prepared compound (a) compared to that of undoped GdAlO3 (b). Plot (c) is the difference spectrum obtained by subtracting the spectrum of the undoped GdAlO3 perovskite from that of the prepared compound. | |
The collected spectrum of the undoped GdAlO3 compound follows closely those reported before.28,29 Assignments are given in Table 4, which includes also the data for the studied Sm3+/Cr3+ doped compound. Compared to the previous investigations,28,29 the present results have allowed to propose assignments for the 4 modes not yet firmly assigned before: the assignment of the Ag mode 6 proposed by Gouadec et al.29 was confirmed to the band now located at 445.0 cm−1 (440 cm−1 as reported before29), and the B1g modes 4 and 6 are assigned to bands observed at 280.0 and 570.0 cm−1, respectively, at frequencies in the ranges observed for other orthorhombic Pbnm aluminium oxide perovskites;29 the B2g mode 1 that had been previously ascribed to the band at 146 cm−1, is now reassigned to the band observed at 119.0 cm−1, while the band at 146 cm−1 is reassigned to the B2g mode 2 (this band is also assigned to the Ag mode 2, following the assignments proposed before;28,29 see Table 4). The assignment of all other modes follows those previously suggested.28,29
Table 4 Assignment of the Raman spectra of GdAlO3 and of Gd0.995Sm0.005Al0.995Cr0.005O3
Symmetry |
Mode |
GdAlO3 |
Gd0.995Sm0.005Al0.995Cr0.005O3 |
This study |
28 |
29 |
This study |
Assigned in 28 to the B2g 1 mode. This band is among all bands the one undergoing the largest frequency shift and broadening, indicating that the movement of the Al3+/Cr3+ ions have a large contribution to the corresponding vibration. The appearance of the additional low-frequency band is, with all probability, a consequence of the Al3+ by Cr3+ substitution. |
Ag |
1 |
94.5 |
95 |
93.5 |
93.0 |
|
2 |
146.5 |
146 |
146 |
145.0 |
|
3 |
233.0 |
232 |
233 |
233.0 |
|
4 |
314.0 |
313 |
315 |
313.0 |
|
5 |
362.0 |
368 |
366 |
361.0b |
|
6 |
445.0 |
— |
440 |
427.0/445.0c |
|
7 |
535.0 |
536 |
534 |
533.5 |
B1g |
1 |
112.0 |
111 |
— |
111.0 |
|
2 |
162.0 |
160 |
— |
159.0 |
|
3 |
222.5 |
222 |
223 |
221.0 |
|
4 |
280.0 |
— |
— |
278.0 |
|
5 |
414.0 |
414 |
405 |
412.0 |
|
6 |
512.0 |
512 |
— |
508.0 |
|
7 |
570.0 |
— |
— |
566.0 |
B2g |
1 |
119.0 |
— |
— |
117.5 |
|
2 |
146.5 |
146a |
146 |
145.0 |
|
3 |
400.0 |
398 |
399 |
399.0 |
|
4 |
468.0 |
475 |
470 |
468.0 |
|
5 |
522.0 |
523 |
522 |
521.0 |
B3g |
1 |
174.0 |
174 |
172 |
172.0 |
|
2 |
217.0 |
217 |
215 |
217.0 |
|
3 |
334.0 |
323 |
— |
334.0 |
|
4 |
400.0 |
400 |
399 |
399.0 |
|
5 |
551.0 |
551 |
— |
547.0 |
The analysis of the Raman spectra provided new data for the undoped material that allowed for fine tuning of the assignments for that material, and are completely new for the synthesized doped material.
3.4 Photoluminescence measurements
3.4.1 Photoluminescence emission spectrum (PL). The room temperature PL spectra of Gd0.995Sm0.005Al0.995Cr0.005O3 and GdAlO3 compounds are shown in Fig. 7. The spectra were collected in time-resolved mode (using a pulsed lamp) with excitation at 377 nm and with a 0.05 ms delay after flash. The intense and well-resolved line observed at 726 nm is ascribed to a Cr3+ 3d–3d transition from the excited 2Eg(2G) to the ground 4A2g(4F) state.30 This emission, with its remarkable tissue-penetrating capability and minimal tissue damage, makes the synthesized compound a promising candidate for in vivo imaging applications and optoelectronic devices.
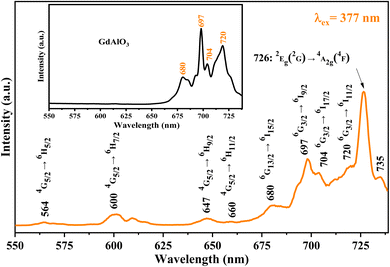 |
| Fig. 7 Comparison between the GdAlO3 and Gd0.995Sm0.005Al0.995Cr0.005O3 PL spectra (collected with excitation at 377 nm). | |
Additionally, an emission line at 735 nm, commonly observed in systems containing high concentrations of Cr3+ ions (e.g., GaAlO3:Cr3+, LaAlO3:Cr3+ (ref. 31)), is likely due to energy transfer between exchange-coupled Cr3+ pairs and individual Cr3+ ions. Emission bands observed at 720, 704, 697, and 680 nm are attributed to the Gd3+ 4f–4f transitions (6Pj → 8S7/2, j = 3/2, 5/2 and 7/2). Bands at 660 nm (4G5/2 → 6H11/2), 647 nm (4G5/2 → 6H9/2), 600 nm (4G5/2 → 6H7/2), and 564 nm (4G5/2 → 6H5/2) are assigned to Sm3+ 4f–4f transitions.32
3.4.2 Photoluminescence excitation spectra (PLE). Fig. 8 presents the PLE spectra of the studied doped material at room temperature, collected with emission at 600 and 697 nm. The spectrum monitored at λem = 697 nm spectra present a narrow UV excitation band corresponding to Gd3+ 4f–4f transition, located at 275 nm and assigned to the absorption 8S7/2 → 6I11/2, and a broad band located at 326 nm, due to the 8S7/2 → 6Pj transition.33 Two additional broad bands in the visible region correspond to Cr3+ 3d–3d transitions: 564 nm (4A2g(4F) → 4T2g(4F)) and 418 nm (4A2g(4F) → 4T1g(4F)).
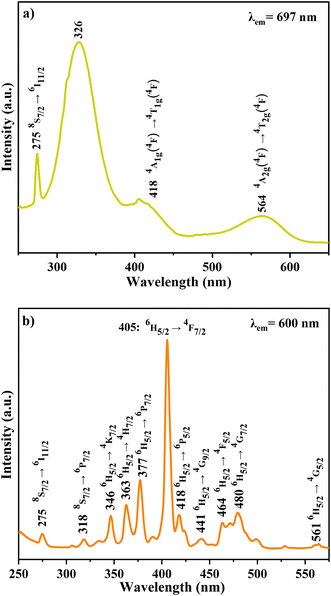 |
| Fig. 8 PLE spectra of the studied doped perovskite monitored at (a) 697 and (b) 600 nm. | |
In contrast, the PLE spectrum monitored at λem = 600 nm reveals multiple excitation bands above 340 nm, arising from intra-configurational 4f–4f transitions of Sm3+.34 These bands, detailed in Table 5, correspond to transitions from the ground state 6H5/2 to various excited states (e.g., 4K7/2, 4H7/2, 6P7/2, 4F7/2, 6P5/2, 4G9/2, 4F5/2, 4G7/2, 4G5/2).35,36 Weak excitation peaks at 275 nm (8S7/2 → 6I11/2) and 318 nm (8S7/2 → 6P7/2) further confirm the occurrence of energy transfer from Gd3+ to Cr3+ ions.
Table 5 Excitation transitions of Gd0.995Sm0.005Al0.995Cr0.005O3
Transition for Sm3+ (ion) |
Wavelength (nm) |
6H5/2 → 4K7/2 |
346 |
6H5/2 → 4H7/2 |
363 |
6H5/2 → 6P7/2 |
377 |
6H5/2 → 4F7/2 |
405 |
6H5/2 → 6P5/2 |
418 |
6H5/2 → 4G9/2 |
441 |
6H5/2 → 4F5/2 |
464 |
6H5/2 → 4G7/2 |
480 |
6H5/2 → 4G5/2 |
561 |
3.4.3 Energy transfer mechanism. The luminescence of Cr3+ can be further enhanced by the presence of lanthanides due to the energy transfer (ET) from Gd3+ and Sm3+ to Cr3+. The photoluminescence (PL) spectrum of GdAlO3:Cr3+ and Sm3+ shows a decrease in the emission intensity of the Gd3+ ion at 697 nm and 720 nm (6PJ → 8S7/2) compared to the emission intensity in the undoped GdAlO3 spectrum (Fig. 7). We also notice that the red line at 726 nm, corresponding to the main luminescence 2Eg(2G) → 4A2g(4F) from GdAlO3:Cr3+ and Sm3+, is intense. This observation confirms the significant energy transfer from Gd3+ and Sm3+ ions to the Cr3+ dopant ions, which further enhances the luminescence of the Cr3+ ions. Energy transfer occurs from the Gd3+ emissions at 697 nm and 720 nm (6PJ → 8S7/2) to the emission of Cr3+ ions. Additionally, energy transfer from Sm3+ emissions at 564 nm (4G5/2 → 6H5/2; green), 600 nm (4G5/2 → 6H7/2; yellow), 647 nm (4G5/2 → 6H9/2; orange), and 660 nm (4G5/2 → 6H11/2; red) further enhances the 2Eg(2G) → 4A2g(4F) emission as shown in Fig. 9. The higher excited states 4T1g(4P), 4T1g(4F), 4T2g(4F) relax non-radiatively to the lowest excited state 2Eg(2G) of Cr3+, which then undergoes radiative relaxation from the 2Eg(2G) state to the ground state 4A2g(4F).
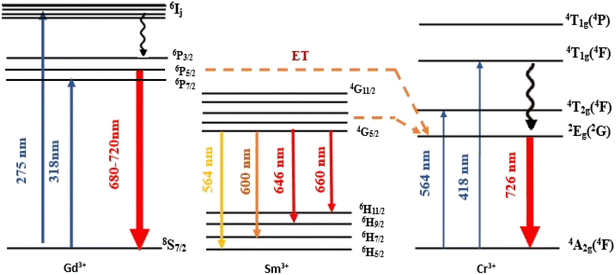 |
| Fig. 9 Energy transfer between Gd3+, Sm3+ and Cr3+ ions. | |
3.4.4 Potential energy surface. In transition ion complexes, electronic transitions are essentially electric dipolar in nature. The d–d transitions for transition metal complexes are in principle prohibited by Laporte's rule.37 Nevertheless, observation of these transitions can be explained by vibronic coupling (coupling between vibrational and electronic wave functions). For example, for an octahedral complex, it can be assumed that some of the vibrations distort the octahedron in such a way that the center of symmetry is destroyed. The states of the configuration dn then no longer strictly preserve their character g and the transitions, normally prohibited by Laporte's rule, become “slightly permitted”.37 Vibronic origins are mainly associated with odd-parity vibrational modes. Table 6 gives the selection rules for the Cr3+ ion occupying an Oh site in the studied doped perovskite. As shown in the table, the Cr3+ 3d–3d transitions observed in the PLE (Fig. 10) are governed by the vibronic mechanism that allow non-graded parity modes (a1u, a2u, t1u, t2u and eu).
Table 6 Selection rules for electronic transitions of Cr3+ occupying an Oh site symmetry in the studied doped perovskite
d–d transitions |
Electric dipole transition (odd vibrational mode that makes the transition permitted) 〈Γi|T1u|Γf〉Γu = A1g |
4A2g(4F) → 4T2g(4F) |
a1u, t1u, t2u, eu |
4A2g(4F) → 4T1g(4F) |
a2u, t1u, t2u, eu |
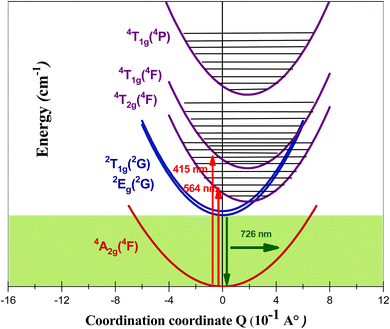 |
| Fig. 10 Adiabatic potential energy curves for 2Eg(2G), 4T2g(4F), 4T1g(4F) and 4T1g(4P) states. | |
To better understand the absorptions and emissions observed in the PLE and PL spectra of the studied compound, the coupled potential energy surface model was used. To simplify the model, a single normal coordination coordinate Q was considered. Due to the characteristics of the electronic transition, which is an intra-configuration d–d excitation, the potential energy minima of the doublet 2Eg(2G) and fundamental 4A2g(4F) states are considered to be at the same configuration (Q = 0 Å) and have the same vibrational frequency. As the metal–ligand antibonding molecular orbitals are populated by d–d excitation, the minimum potential energy of the states 4T2g(4F), 4T1g(4F) and 4T1g(4P) is shifted by the quantity ΔQ relative to the minimum of the 2Eg(2G) state.
The potentials for the excited states 2Eg(2G), 4T2g(4F), 4T1g(4F) and 4T1g(4P) are as follows:
|
V(2Eg(2G)) = 1/2(kQ2) + EZPL(2Eg(2G))
| (1a) |
|
V(4T2g(4F)) = 1/2(k(Q − ΔQ1)2) + EZPL(4T2g(4F))
| (1b) |
|
V(4T1g(4F)) = 1/2(k(Q − ΔQ1)2) + EZPL(4T1g(4F))
| (1c) |
|
V(4T1g(4P)) = 1/2(k(Q − ΔQ1)2) + EZPL(4T1g(4P))
| (1d) |
where
EZPL(
2E
g(
2G)),
EZPL(
4T
2g(
4F)),
EZPL(
4T
1g(
4F)), and
EZPL(
4T
1g(
4P)) are the energies of the minima for the
2E
g(
2G),
4T
2g(
4F),
4T
1g(
4F) and
4T
1g(
4P) states, respectively, and
k is the Raman frequency of GdAlO
3 assigned to the ground and excited states. The adiabatic potential state surfaces, obtained from
eqn (1) are depicted in
Fig. 10.
3.4.5 Crystal field analysis of Cr3+ in GdAlO3 and relevance to PLE and PL spectra. The electronic structure of Cr3+ in GdAlO3 can be ascertained by employing the Crystal Field Theory (CFT). In this work, we expand on previous studies by providing a more detailed analysis of the crystal field effects and their influence on the photoluminescence (PL) behavior of Cr3+ in this host material. The Cr3+ ion energy levels are assessed using a complete Hamiltonian, and the crystal field strength (Dq), as well as the Racah parameters (B and C), are found by analyzing the optical PLE and PL spectra.The Cr3+ ion energy levels are assessed using the complete Hamiltonian:38–50
|
H = H0 + Hee(B, C) + HTrees(αTrees) + HCF(Dq) + HSO(ζ)
| (2) |
where
H0 is the configuration Hamiltonian,
Hee is the electron–electron repulsion Hamiltonian,
51–53 HSO is the spin–orbit coupling Hamiltonian, the
HTrees Hamiltonian included the Trees correction,
54,55 and
HCF is the crystal field Hamiltonian for octahedral symmetry (
Oh):
56–58 |
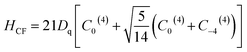 | (3) |
In
eqn (2) and
(3) the parameter
Dq represents the ligand field splitting, while numerical techniques are used to specifically calculate the matrix elements for the
Cq(k) operators, as described in ref.
59. The
αTrees and
ζ parameters are calculated using the following equations:
54–56and
with:
|
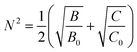 | (5) |
where,
N is the reduction factor.
60
We derived a stable crystal-field and Racah parameters for Cr3+ ions, which allow to calculate the electronic energy levels of Cr3+(3d3) ions in the Oh symmetry site of a Cr3+ doped GdAlO3 perovskite (GdAlO3:Cr3+). The free ion Cr3+ parameters B0, C0, α0 and ζ0 are listed in Table 7.41–43 The crystal-field strength parameter Dq was computed using the energy of the quartet excited state 4T2g(4F), and the Racah parameters B and C were calculated based on the observed excited states of the 4T1g(4F) quartet and the 2Eg(2G) doublet, decreasing in relation to their values for the free ion due to the covalency effect. The values of α and ζ were obtained using eqn (3)–(5). The calculations were done using a custom code that was developed in our laboratory, by S. Kammoun, using the Maple 2024 software. The calculated energies (Table 8) are in excellent agreement with the experimental findings of Yeung et al.,61 thus validating our computational method.
Table 7 Optical parameter values for the theoretical study of GdAlO3:Cr3+
Parameter |
Value [this study] |
Parameter |
Value43,51 |
Dq (cm−1) |
1773 |
B0 (cm−1) |
918 |
B (cm−1) |
617 |
C0 (cm−1) |
4133 |
C (cm−1) |
3045 |
α0 |
30 |
α (cm−1) |
21.12 |
ζ0 |
275 |
ζ (cm−1) |
230.75 |
|
|
Dq/B |
2.87 |
|
|
C/B |
4.94 |
|
|
Table 8 Experimental and calculated energies (cm−1) of GdAlO3:Cr3+
Electrons are usually transferred from one atom to another during ionic bonding, producing positively and negatively charged ions. Eqn (6) allows to evaluate the type of bonding for Cr3+ in GdAlO3:Cr3+.62 In this equation, h is a measure of the degree of electron delocalization between the cation to the ligands. For the considered system, the constant k is equal to 0.21.63,64
|
 | (6) |
The calculated large value for h (1.44) indicates a high degree of d-electron delocalization, revealing the high ionic character of the bonds established between Cr3+ ions and their ligands.
The basic optical characteristics of 3dn transition-metal ions doped into crystalline hosts can be explained by the crystal-field model. The Tanabe–Sugano energy-level diagram presented in Fig. 11 shows how the ratio Dq/B affects the electronic energy levels of 3dn trivalent ionic species in the octahedral site of the GdAlO3 host. The vertical line in Fig. 11 for Dq/B = 2.87 corresponds to Cr3+ doped into the GdAlO3 perovskite in an octahedral site symmetry.
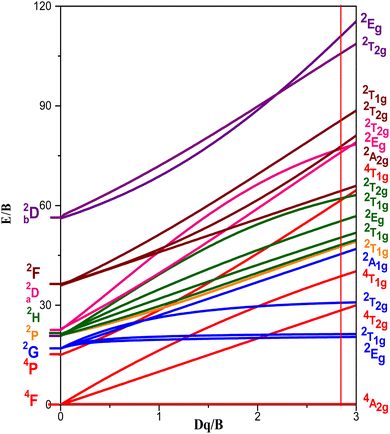 |
| Fig. 11 Tanabe–Sugano energy-levels diagram highlighting the case of Cr3+(3d3) ion doped into the octahedral symmetry site of GdAlO3, as expressed by the vertical line for Dq/B = 2.87. | |
The theoretical study for determining the electronic structure of Cr3+ ion in GdAlO3:Cr,Sm using crystal field theory confirms that this ion replaces Al3+ at an octahedral site. Indeed, there is a satisfactory agreement between the experimental and theoretical levels, considering that Cr3+ replaces Al3+ at an octahedral site. The importance of the theoretical study using crystal field theory lies in the fact that it confirms the oxidation state and the site occupied by the Cr3+ ion. Also, it is only through this theoretical study that the d–d transitions can be accurately assigned, as these transitions vary for the same ion depending on the matrix (intermediate crystal field).
4. Conclusion
In conclusion, the orthorhombic Gd0.995Sm0.005Al0.995Cr0.005O3 sample, synthesized through the solid-state reaction method, underwent comprehensive investigation of its structure, morphology, and photoluminescence properties. The material crystallized in the orthorhombic Pbnm space group, with Cr3+ ions substituting for Al3+ ions in octahedral site symmetry within the GdAlO3:Sm3+, Cr3+ matrix. Examination via Scanning Electron Microscopy (SEM) and Energy Dispersive X-ray analysis confirmed the morphology and homogeneity of the sample's chemical composition. Photoluminescence excitation (PLE) and photoluminescence (PL) spectra revealed the electronic structure of Cr3+ ions in the Oh symmetry site, with theoretical studies allowing for precise attribution of their 3d–3d transitions. Additionally, intra-configurational 4f–4f transitions of Sm3+ resulted in various excitation bands observed in the PLE spectrum at higher wavelengths. Moreover, the photoluminescence studies give additional support to the occurrence of energy transfer among Gd3+, Sm3+, and Cr3+ ions. Based on these findings, the synthesized nanophosphor samples demonstrate strong potential for applications in solid-state lighting.
Data availability
Data are available upon request to the authors.
Conflicts of interest
The authors declare that they have no known competing financial interests or personal relationships that could have appeared to influence the work reported in this paper.
Acknowledgements
The CQC-IMS is supported by FCT through projects UI0313B/QUI/2020, UI0313P/QUI/2020 and LA/P/0056/2020 (national funds). This work was also supported by FCT – Fundação para a Ciência e Tecnologia, I. P. through the projects UIDB/04564/2020 and UIDP/04564/2020, with DOI identifiers 10.54499/UIDB/04564/2020 and 10.54499/UIDP/04564/2020, respectively. Access to TAIL-UC facility funded under QREN-Mais Centro Project No. ICT_2009_02_012_1890 is gratefully acknowledged.
References
- R. E. Rojas-Hernandez, F. Rubio-Marcos, M. A. Rodriguez and J. F. Fernandez, Long lasting phosphors: SrAl2O4:Eu, Dy as the most studied material, Renewable Sustainable Energy Rev., 2018, 81, 2759–2770 CrossRef.
- D. Singh, V. Tanwar, S. Bhagwan and I. Singh, Recent advancements in luminescent materials and their potential applications, Adv. Magn. Opt. Mater., 2016, 317–352 Search PubMed.
- P. Kumar, D. Singh, I. Gupta, S. Singh, V. Kumar, H. Kumar and S. K. Chhikara, Cool green light emitting GdAlO3:Tb3+ perovskite nanomaterials: crystal structure and spectroscopic characteristics for advance display appliances, Inorg. Chem. Commun., 2022, 154, 110064 CrossRef.
- I. Gupta, S. Singh, S. Bhagwan and D. Singh, Rare earth (RE) doped phosphors and their emerging applications: a review, Ceram. Int., 2021, 47, 19282–19303 CrossRef.
- P. Kumar, D. Singh, I. Gupta, S. Singh, V. Kumar, H. Kumar and S. K. Chhikara, Perovskite GdAlO3:Dy3+ nanophosphors: a gel-combustion synthesis, phase evaluation and down conversion luminescent characteristics for lighting applications, J. Lumin., 2022, 252, 119409 CrossRef.
- K. M. Girish, S. C. Prashantha and H. Nagabhushana, Facile combustion based engineering of novel white light emitting Zn2TiO4:Dy3+ nanophosphors for display and forensic applications, J. Sci.:Adv. Mater. Devices, 2017, 2, 360–370 Search PubMed.
- P. Kumar, S. Singh, I. Gupta, V. Kumar and D. Singh, Luminous LaAlO3:Dy3+ perovskite nanomaterials: synthesis, structural and luminescent characteristics for WLEDs, Luminescence, 2022, 37, 1932–1941 CrossRef CAS PubMed.
- E. Pavitra, G. S. R. Raju, G. L. Varaprasad, N. R. Chodankar, M. V. B. Rao, N. M. Rao, J. Y. Park, Y.-K. Han and Y. S. Huh, Desired warm white light emission from a highly photostable and single-component Gd2TiO5:Dy3+/Eu3+ nanophosphors for indoor illuminations, J. Alloys Compd., 2021, 875, 160019 CrossRef CAS.
- P. Kumar, S. Singh, I. Gupta, V. Kumar and D. Singh, Preparation and luminescence behaviour of perovskite LaAlO3:Tb3+ nanophosphors for innovative displays, Optik, 2022, 267, 169709 CrossRef CAS.
- P. Kumar, S. Singh, I. Gupta, A. Dalal, V. Kumar and D. Singh, Preparation, structural and photometric properties of single-phased Gd3Al5O12:Tb3+ green-emitting phosphors for solid state lighting purpose, Mater. Sci. Eng., B, 2023, 288, 116189 CrossRef CAS.
- V. Tanwar, S. Singh, I. Gupta, P. Kumar, H. Kumar, B. Mari and D. Singh, Preparation and luminescence characterization of Eu(III)-activated Forsterite for optoelectronic applications, J. Mol. Struct., 2022, 1250, 131802 CrossRef CAS.
- I. Gupta, P. Kumar, S. Singh, S. Bhagwan, S. K. Chhikara and D. Singh, Synthesis, structural and optical investigations of YAlO3:Er3+ perovskites for near UV pumped photonic appliances, Inorg. Chim. Acta, 2022, 543, 121183 CrossRef CAS.
- D. Singh, S. Sheoran and J. Singh, Optical characterization of Eu3+ doped MLSiO4 (M = Ca, Sr, Ba and L = Mg) phosphor materials for display devices, J. Mater. Sci.: Mater. Electron., 2018, 29, 294–302 CrossRef CAS.
- I. Gupta, D. Singh, S. Singh, P. Kumar, S. Bhagwan and V. Kumar, Study of structural and spectroscopic characteristics of novel color tunable yellowish-white Dy3+ doped Gd4Al2O9 nanophosphors for NUV-based WLEDs, J. Mol. Struct., 2022, 1272, 134199 CrossRef.
- Y. Wu, X. Yin, Q. Zhang, W. Wang and X. Mu, The recycling of rare earths from waste tricolor phosphors in fluorescent lamps: a review of processes and technologies, Resour., Conserv. Recycl., 2014, 88, 21–31 CrossRef.
- I. Gupta, D. Singh, S. Singh, P. Kumar, S. Bhagwan and V. Kumar, Phase recognition and spectroscopic characteristics of single-phase Tb3+ doped Gd4Al2O9 nanophosphors for NUV energized advanced photonic appliances, J. Lumin., 2022, 252, 119327 CrossRef CAS.
- R. K. Sajwan, S. Tiwari, T. Harshit and A. K. Singh, Recent progress in multicolor tuning of rare earth-doped gadolinium aluminate phosphors GdAlO3, Opt. Quantum Electron., 2017, 49, 344 CrossRef.
- J. I. Eldridge, Single fiber temperature probe configuration using anti-Stokes luminescence from Cr:GdAlO3, Meas. Sci. Technol., 2018, 29, 065206 CrossRef.
- S. Adachi, Review – Photoluminescence properties of Cr3+-activated oxide phosphors, ECS J. Solid State Sci. Technol., 2021, 10, 026001 CrossRef.
- P. Dorenbos, E. Bougrine, J. D. Haas, C. Eijk and M. Korzhik, Scintillation properties of GdAlO3:Ce crystals, Radiat. Eff. Defects Solids, 1995, 135, 321–323 CrossRef.
- M. Y. Gneber, I. Elhamdi, J. Messoudi, R. Dhahri, F. Sahnoune, M. Jemmali, M. Hussein, E. Dhahri and B. F. O. Costa, Investigation of variable range hopping and dielectric relaxation in GdCrO3 orthochromite perovskites, RSC Adv., 2024, 14, 36161–36172 RSC.
- K. Momma and F. Izumi, VESTA 3 for three-dimensional visualization of crystal, volumetric and morphology data, J. Appl. Crystallogr., 2011, 44, 1272–1276 CrossRef.
- I. Elhamdi, H. Souissi, O. Taktak, S. Kammoun, E. Dhahri, J. Pina, B. F. O. Costa and E. López-Lago, Optical characterization and defect-induced behavior in ZnAl1.999Ho0.001O4 spinel: unraveling novel insights into structure, morphology, and spectroscopic features, Heliyon, 2024, 10, E29241 CrossRef PubMed.
- I. Elhamdi, F. Mselmi, H. Souissi, S. Kammoun, E. Dhahri, P. Sanguino and B. F. O. Costa, Summerfield scaling model and electrical conductivity study for understanding transport mechanisms of a Cr3+ substituted ZnAl2O4 ceramic, RSC Adv., 2023, 13, 3377–3393 RSC.
- F. Mselmi, I. Elhamdi, M. Bejar and E. Dhahri, Cross-relaxation induced efficient 1.55 μm emission in La1.95Er0.05Ti2O7 towards an application as an amplifier for silica-fibers, Opt. Mater., 2023, 137, 113555 CrossRef.
- C. S. McCamy, Correlated color temperature as an explicit function of chromaticity coordinates, Color. Res. Appl., 1992, 17, 142–144 CrossRef.
- S. Sun and Q. Xu, Fabricating a novel intragranular microstructure for Al2O3/GdAlO3 ceramic composites, Materials, 2018, 11, 1879 CrossRef PubMed.
- A. Chopelas, Single-crystal Raman spectra of YAlO3 and GdAlO3: comparison to several orthorhombic ABO3 perovskites, Phys. Chem. Miner., 2011, 38, 709–726 CrossRef.
- G. Gouadec, P. Colomban, N. Piquet, M. F. Trichet and L. Mazerolles, Raman/Cr3+ fluorescence mapping of a melt-grown Al2O3/GdAlO3 eutectic, J. Eur. Ceram. Soc., 2005, 25, 1447–1453 CrossRef.
- G. Wei, P. Li, R. Li, Y. Wang, S. He, J. Li, Y. Shi, H. Suo, Y. Yang and Z. Wang, How to Achieve Excellent Luminescence Properties of Cr Ion-Doped Near-Infrared Phosphors, Adv. Opt. Mater., 2023, 11, 2301794 CrossRef CAS.
- H. Luo and P. Dorenbos, The dual role of Cr3+ in trapping holes and electrons in lanthanide co-doped GdAlO3 and LaAlO3, J. Mater. Chem. C, 2018, 6, 4977–4984 RSC.
- N. Wantana, S. Kaewjaeng, S. Kothan, H. J. Kim and J. Kaewkhao, Energy transfer from Gd3+ to Sm3+ and luminescence characteristics of CaO–Gd2O3–SiO2–B2O3 scintillating glasses, J. Lumin., 2017, 181, 382–386 CrossRef CAS.
- J. Li, J. G. Li, J. Li, S. Liu, X. Li, X. Sun and Y. Sakka, Development of Eu3+ activated monoclinic, perovskite, and garnet compounds in the Gd2O3–Al2O3 phase diagram as efficient red-emitting phosphors, J. Solid State Chem., 2013, 206, 104–112 CrossRef CAS.
- R. Cao, M. Wu, B. Lan, T. Huang, J. Nie, F. Cheng, X. Luo and J. Wang, Study on the properties of Sm3+-Doped CaTbAl3O7 phosphors, J. Lumin., 2025, 277, 120898 CrossRef CAS.
- P. Kumar, D. Singh, I. Gupta, S. Singh and V. Kumar, Structural and luminescent characteristics of orthorhombic GdAlO3:Sm3+ nanocrystalline materials for solid state lighting, Chem. Phys. Lett., 2023, 812, 140277 CrossRef CAS.
- P. Kumar, D. Singhlogo and I. Gupta, Gadolinium-based Sm3+ activated GdSr2AlO5 nanophosphor: synthesis, crystallographic and opto-electronic analysis for warm LEDs, RSC Adv., 2023, 13, 7703–7718 RSC.
- O. Kahn, Structure electrónique des eléments de transitions, Presses Universitaires de France, Paris, 1997 Search PubMed.
- O. Taktak, H. Souissi and S. Kammoun, Optical absorption properties of ZnF2-RO-TeO2 (R = Pb, Cd and Zn) glasses doped with chromium (III): Neuhauser model and crystal field study, Opt. Mater., 2021, 113, 110682 CrossRef CAS.
- H. Souissi, O. Taktak and S. Kammoun, Crystal field study of chromium (III) ions doped antimony phosphate glass: Fano's antiresonance and Neuhauser models, Indian J. Phys., 2018, 92, 1153–1160 CrossRef CAS.
- O. Maalej, O. Taktak, B. Boulard and S. Kammoun, Study with analytical equations of absorption spectra containing interference dips in fluoride glasses doped with Cr3+, J. Phys. Chem. B, 2016, 120, 7538–7545 CrossRef CAS PubMed.
- S. Kammoun and J. El Ghoul, Structural and optical investigation of Co-doped ZnO nanoparticles for nano optoelectronic devices, J. Mater. Sci.: Mater. Electron., 2021, 32, 7215–7225 CrossRef CAS.
- A. Neffati, H. Souissi and S. Kammoun, Electronic structure of Co-doped ZnO nanorods, J. Appl. Phys., 2012, 112, 083112 CrossRef.
- F. Mselmi, A. Neffatiand and S. Kammoun, Theoretical investigation of the cathodoluminescence spectra of Co-doped ZnO nanowires, J. Lumin., 2018, 198, 124–131 CrossRef CAS.
- F. Mselmi, O. Taktak, H. Souissi and S. Kammoun, Correlation between experimental spectroscopic study and crystal-field calculations of Co2+ ions in α-ZnAl2S4 spinel, J. Lumin., 2019, 206, 319–325 CrossRef CAS.
- I. Elhamdi, H. Souissi, S. Kammoun, E. Dhahri, J. Pina, B. F. O. Costa and E. López-Lago, Comprehensive characterization and optoelectronic significance of Ho3+ and Cr3+ Co-doped ZnAl2O4 spinels, Dalton Trans., 2024, 53, 7721–7733 RSC.
- I. Elhamdi, H. Souissi, S. Kammoun, E. Dhahri, A. L. B. Brito, R. Fausto and B. F. O. Costa, Experimental determination and modeling of structural, vibrational and optical properties of the ZnAl2−xCrxO4 (x = 0 and 0.05) spinels, J. Lumin., 2023, 263, 119968 CrossRef.
- I. Elhamdi, F. Mselmi, S. Kammoun, E. Dhahri, A. J. Carvalho, P. Tavares and B. F. O. Costa, A far-red-emitting ZnAl1.95Cr0.05O4 phosphor for plant growth LED applications, Dalton Trans., 2023, 52, 9301–9314 RSC.
- O. Taktak, H. Souissi, I. Elhamdi, A. Oueslati, S. Kammoun, M. Gargouri and E. Dhahri, Optical investigations and theoretical simulation of organic-inorganic hybrid: TPA-CoCl4, Opt. Mater., 2024, 150, 115251 CrossRef.
- H. Souissi, S. Kammoun, E. Dhahri and E. López-Lago, Optical and theoretical study of NaCr(P2O7): a look through the Neuhauser model and Racah theory, Dalton Trans., 2024, 53, 14422–14432 RSC.
- H. Souissi, S. Kammoun, E. Dhahri, E. López-Lago and B. F. O. Costa, Exploring the structural and optical properties of lithium-chromium phosphate Li3Cr2(PO3)4, Heliyon, 2024, 10, e36188 CrossRef PubMed.
- S. Sugano, Y. Tanabe and H. Kamimura, Multiplets of Transition-Metal Ions in Crystals, Academic Press, New York, USA, 1970 Search PubMed.
- J. S. Griffith, The Theory of Transition-Metal Ions, Cambridge University Press, Cambridge, UK, 1961 Search PubMed.
- R. C. Powell, Physics of Solid-State Laser Materials, Springer-Verlag, New York, USA, 1st edn, 1998, pp. 215–233 Search PubMed.
- Z.-Y. Yang, C. Rudowicz and Y.-Y. Yeung, Microscopic spin-Hamiltonian parameters and crystal field energy levels for the low C3 symmetry Ni2+ centre in LiNbO3 crystals, Phys. B, 2004, 348, 151–159 CrossRef.
- C. Rudowicz, Z.-Y. Yang, Y.-Y. Yeung and J. Qin, Crystal field and microscopic spin Hamiltonians approach including spin–spin and spin–other-orbit interactions for d2 and d8 ions at low symmetry C3 symmetry sites: V3+ in Al2O3, J. Phys. Chem. Solids, 2003, 64, 1419–1428 CrossRef.
- D. J. Newman, B. Ng, Crystal Field Handbook, Cambridge University Press, Cambridge, UK, 1st edn, 2000, pp. 28–36 Search PubMed.
- B. G. Wybourne, Spectroscopic Properties of Rare Earth, Wiley, New York, USA, 1965, 1st edn, pp. 48–234 Search PubMed.
- I. Elhamdi, H. Souissi, O. Taktak, J. Elghoul, S. Kammoun, E. Dhahri and B. F. Costa, Experimental and modeling study of ZnO: Ni nanoparticles for near-infrared light emitting diodes, RSC Adv., 2022, 12, 13074–13086 RSC.
- J. P. Elliott, B. R. Judd and W. A. Runciman, Energy levels in rare-earth ions, Proc. R. Soc. London, Ser. A, 1957, 240, 509–523 Search PubMed.
- M. G. Zhao, J. A. Xu, G. R. Bai and H. S. Xie, d-orbital theory and high-pressure effects upon the EPR spectrum of ruby, Phys. Rev. B:Condens. Matter Mater. Phys., 1983, 27, 1516–1522 CrossRef.
- Y. Y. Yeung and C. Rudowicz, Ligand field analysis of the 3dN ions at orthorhombic or higher symmetry sites, Comput. Chem., 1992, 16, 207–216 CrossRef.
- W. Seeber, D. Ehrt and H. Eberdorff-Heidepriem, Spectroscopic and laser properties of Ce3+/Cr3+/Nd3+ co-doped fluoride phosphate and phosphate glasses, J. Non-Cryst. Solids, 1994, 171, 94–104 CrossRef.
- C. K. Jorgensen, Absorption Spectra and Chemical Bonding in Complexes, Pergamon Press, London, UK, 1963, p. 113 Search PubMed.
- V. Mahalingam and J. Thirumalai, Effect of co-doping of alkali metal ions on Ca0.5RE1−x(MoO4)2:xEu3+ (RE = Y, La) phosphors with enhanced luminescence properties, RSC Adv., 2016, 6, 80390–80397 RSC.
|
This journal is © The Royal Society of Chemistry 2025 |
Click here to see how this site uses Cookies. View our privacy policy here.