DOI:
10.1039/C6RA03023H
(Paper)
RSC Adv., 2016,
6, 32653-32660
The first report on the eco-friendly synthesis of 5-substituted 1H-tetrazoles in PEG catalyzed by Cu(II) immobilized on Fe3O4@SiO2@L-arginine as a novel, recyclable and non-corrosive catalyst
Received
1st February 2016
, Accepted 21st March 2016
First published on 24th March 2016
Abstract
Cu(II) immobilized on Fe3O4@SiO2@L-aginine as a novel, inexpensive and non-corrosive nanostructured compound catalyzed the cycloaddition reaction of various organic nitriles with sodium azide. This combination leads to obtain a series of 5-substituted-1H-tetrazoles under mild reaction conditions in polyethylene glycol (PEG). The structure of the catalyst was studied by Fourier transform infrared spectroscopy (FT-IR), scanning electron microscopy (SEM), X-ray diffraction (XRD) thermogravimetric analysis (TGA) and atomic absorption spectroscopy (AAS). Ease of operation, high efficiency, low cost, recovery, and reusability for four continuous cycles are the noteworthy features of the currently employed heterogeneous catalytic system.
1 Introduction
Tetrazoles are a class of heterocycles with a wide range of applications that are receiving considerable attention in the recent years.1 Tetrazoles are nitrogen-rich compounds, which can be used in a wide range of applications, such as in material sciences, catalysis technology, information recording systems, explosives, and possible application in high energy chemistry.2 Recently, tetrazole moieties were widely used for binding aryl thiotetrazolylacetanilides with HIV-1 reverse transcriptase.3 Also they form stable complexes with metals4 and have been used as ligands for palladium-catalyzed reactions.5 Because of their potent usefulness, the various methods for the synthesis of tetrazole frameworks are well documented in the literature. In general, the most direct and versatile method for the synthesis of 5-substituted 1H-tetrazoles is [2 + 3] cycloaddition of nitriles and an azide moiety under the influence of appropriate catalyst and different solvent conditions. Several new catalysts have been investigated, such as zinc salts,6 β-cyclodextrin,7 Fe(OAc)2,8 CdCl2,9 AgNO3,10 SiO2–H3BO3,11 Cu-MCM-41,12 and copper triflates.13 Although all of these methods are beneficial, a number of them have one or more of the following drawbacks: the use of DMF as solvent, tedious workup of the reaction mixture, refluxing for a prolonged period of time, toxic metals and difficulty in separation and recovery of the catalyst.14 Hence, the choice of a suitable catalyst and environmentally benign method that avoids all of these drawbacks is of key importance to get good results. In the other hand, magnetic nano-materials showed major impacts on catalysis and many other areas, such as medicine, drug delivery, and remediation.15 There is an ever increasing interest in the synthesis, characterization and surface modification of magnetic nanoparticles because of their potential applications in biotechnology, biomedicine, environmental, material science and catalysis.16 Currently, much attention is focused on the synthesis of magnetic core shell structures by coating a SiO2 shell around magnetic nanoparticles.17 Silica coating endows the magnetic nanoparticles with easily modifiable surface, which may be used to graft various desirable functional groups. Metal nanoparticles (M-NPs) are of significant interest for technological applications in several areas of science and industry, especially in catalysis due to their high activity. Our research group devoted particular notice to the progress of environmentally friendly and efficient process to minimize waste production in chemical manufacture. Hence, as a part of our research program directed toward the utility of magnetic nanoparticles,18–20 herein we report the preparation of Cu(II) immobilized on Fe3O4@SiO2@L-arginine as a novel and heterogeneous catalyst for the preparation of 1H-tetrazoles in polyethylene glycol (PEG).
2 Results and discussion
In this research we describe the preparation of a novel magnetic nanoparticle catalyst, by anchoring L-arginine on the surface of silica-coated iron oxide nanoparticles and then treatment of Fe3O4@SiO2@L-arginine with Cu(NO3)2·3H2O, which leads to immobilized Cu(II) on Fe3O4@SiO2@L-arginine (Scheme 1).
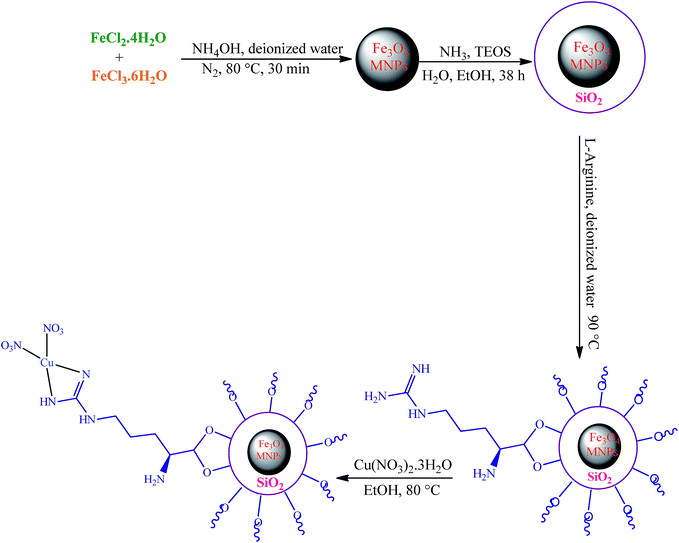 |
| Scheme 1 General route for the synthesis of Cu(II) immobilized on Fe3O4@SiO2@L-arginine. | |
2.1 Characterization
Successful functionalization of MNPs can be verified by the FT-IR spectra, which has been shown in Fig. 1. The presence of a peak around 2856–2924 cm−1 corresponding to the aliphatic C–H stretching vibration of the methylene group.16,17 The FT-IR measurement of Fe3O4@L-arginine shows the stretching vibration of Fe–O and C
O bonds at 583 and 1633 cm−1, respectively. It means that the L-arginine was supported on the magnetite surface. FT-IR spectrum of the Cu(II) immobilized on Fe3O4@SiO2@L-arginine showed the expected bands, including a distinctive band due to C
N stretching vibration, which appears at low frequency compared to Fe3O4@SiO2@L-arginine.
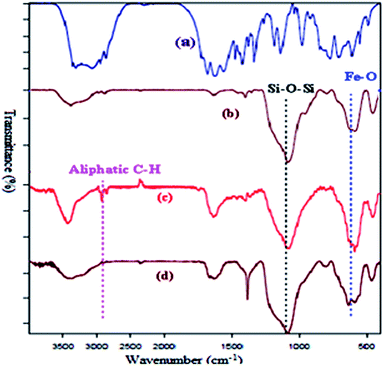 |
| Fig. 1 FT-IR spectra of pure L-arginine (a), Fe3O4@SiO2 (b) Fe3O4@SiO2@L-arginine (c) and Cu(II) immobilized on Fe3O4@SiO2@L-arginine (d). | |
Fig. 2 shows scanning electron microscopy (SEM) images of the Cu(II) immobilized on Fe3O4@SiO2@L-arginine nanoparticles. According to Fig. 2, nanoparticles were obtained with approximately spherical shape.
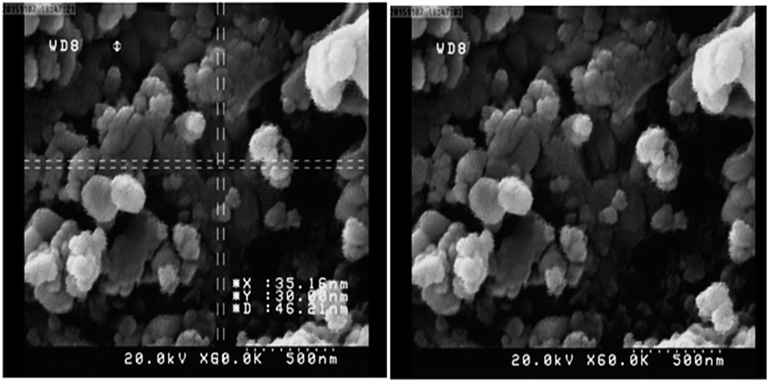 |
| Fig. 2 SEM image of Cu(II) immobilized on Fe3O4@SiO2@L-arginine. | |
The structure and phase purity of Cu(II) immobilized on Fe3O4@SiO2@L-arginine NPs was studied by X-ray diffraction analysis (XRD), (Fig. 3) The results are in agreement with standard patterns of inverse cubic spinel magnetite (Fe3O4) crystal structure, which shows diffraction peaks; corresponding to (2 2 0), (3 1 1), (4 0 0), (4 2 2), (5 1 1), and (4 4 0).17
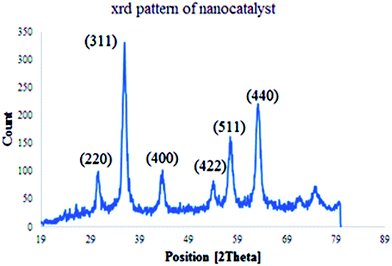 |
| Fig. 3 The X-ray diffraction pattern of Cu(II) immobilized on Fe3O4@SiO2@L-arginine nanoparticle. | |
The thermal behavior of Cu(II) immobilized on Fe3O4@SiO2@L-arginine was investigated by thermogravimetric analysis in air from room temperature to 800 °C (TGA) (Fig. 4). The TGA was used to determine the percent of chemisorbed organic functional groups on the surface of magnetic nanoparticle. The weight loss below 200 °C for the sample is due to loss of adsorbed water, solvents and surface hydroxyl groups. Complete loss of organic moieties of the grafted L-arginine@Cu(II) immobilized on Fe3O4@SiO2 was seen in the temperature range from 250 to 450 °C, and the amount of organic component was found to be about 20% of the total solid catalyst. Thus, the catalyst was stable to 250 °C and could be used in organic reactions at temperatures in the range of 100–120 °C. Differential thermogravimetric analysis (DTG) of catalyst reveals that the fastest weight loss occurred approximately between 400 °C and 450 °C.
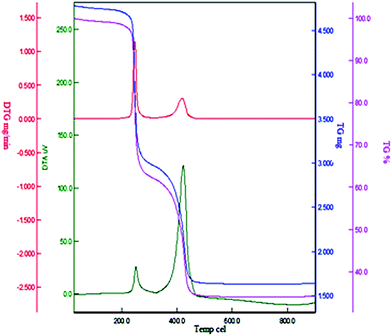 |
| Fig. 4 TGA curve of Cu(II) immobilized on Fe3O4@SiO2@L-arginine. | |
Atomic absorption spectroscopy (AAS) was employed to determine the exact amount of copper in Cu(II) immobilized on Fe3O4@SiO2@L-arginine that was found to be 3.4 × 10−4 mol g−1.
2.2 Catalytic activity of Cu(II) immobilized on Fe3O4@SiO2@L-arginine
The catalytic activity of this nanocatalyst was examined in the [2 + 3] cycloaddition reaction of various organic nitriles with sodium azide, which yielded the corresponding 5-substituted-1H-tetrazoles, as sole product (Scheme 2).
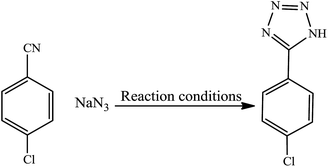 |
| Scheme 2 Preparation of 5-(4-chlorophenyl)-1H-tetrazole. | |
In order to obtain the best conditions for the synthesis of described heterocyclic compounds, the reaction of 4-chlorobenzonitrile and sodium azide was selected as a model reaction and was studied in the presence of Cu(II) immobilized on Fe3O4@SiO2@L-arginine. Initially, the influence of different reaction parameters (including solvent, temperature and amount of catalyst) on the outcome of reaction was evaluated. The solvent has a prominent influence on the yield of product. The experimental results shown that among the tested solvents, PEG is the best media for the synthesis of tetrazole (Table 1, entry 3) and was used in subsequent optimization studies. The reaction in DMF and DMSO was not completed and further increase in the reaction time has no significant effect on the yield of product (Table 1, entries 1 and 2). Also other solvents are not suitable for this reaction. The effect of temperature was investigated by carrying out the model reaction at different temperatures in PEG (room temperature, 70, 100 and 120 °C, Table 1, entries 3, 5, 6 and 7), the best results were obtained at 120 °C. The effect of the amount of catalyst on the outcome of the reaction was also evaluated that the best result was obtained by 0.030 g of catalyst. However, using higher amount of catalyst no significant effect on reaction rate was observed and the same isolated yield of product was obtained (Table 1, entry 10), while a decrease in the amount of catalyst decreases the tetrazole yield. In a blank reaction, the synthesis of 5-(4-chlorophenyl)-1H-tetrazole was performed in the absence of catalyst; which the product was obtained in low yield after 3 h thus the presence of catalyst is vital for the synthesis of tetrazole compounds in short reaction time (Table 1, entry 8). Therefore, the optimized reaction conditions for the synthesis of 5-substituted 1H-tetrazoles, which has been obtained was: PEG as solvent, 0.030 g of Cu(II) immobilized on Fe3O4@SiO2@L-arginine and 120 °C (Table 1, entry 3).
Table 1 Screening of reaction parameters for the formation of 5-(4-chlorophenyl)-1H-tetrazole
Entry |
Solvent |
Temperature (°C) |
Cat. (g) |
Time (h) |
Yielda (%) |
Isolated yield. Reaction in the presence of Fe3O4@SiO2. Reaction in the presence of Fe3O4@SiO2@L-arginine. |
1 |
DMSO |
120 |
0.030 (1.020 mol%) |
3 |
80 |
2 |
DMF |
120 |
0.030 (1.020 mol%) |
3 |
48 |
3 |
PEG |
120 |
0.030 (1.020 mol%) |
3 |
95 |
4 |
EtOH |
80 |
0.030 (1.020 mol%) |
3 |
N.R. |
5 |
PEG |
100 |
0.030 (1.020 mol%) |
3 |
74 |
6 |
PEG |
70 |
0.030 (1.020 mol%) |
3 |
15 |
7 |
PEG |
r.t. |
0.030 (1.020 mol%) |
3 |
N.R. |
8 |
PEG |
120 |
0.000 |
3 |
35 |
9 |
PEG |
120 |
0.010 |
3 |
61 |
10 |
PEG |
120 |
0.050 |
2.5 |
95 |
11b |
PEG |
120 |
0.030 |
3 |
35 |
12c |
PEG |
120 |
0.030 |
3 |
36 |
In order to extend the scope of our methodology for the synthesis of 5-substituted 1H-tetrazoles, we next used different nitriles for this cycloaddition reaction (Scheme 3, Table 2). The activity of nitrile compound towards azide ion plays an important role in this cycloaddition reaction. Among the various nitrile compounds, electron-withdrawing groups reacted faster than electron-donating groups and give excellent yields in shorter reaction times (Table 2, entries 1–8). However, when a nitrile bearing an electron-donating group is used (Table 2, entries 9 and 11), the corresponding tetrazole is obtained in moderate to good yields with a prolonged reaction time because the electron donating properties of this group deactivated the nitrile group for [2 + 3] cycloaddition reaction, thus the reaction time is longer comparing with substrates with electron withdrawing groups. It is noteworthy that aryl dicyano derivatives such as terephthalonitrile also reacted smoothly with sodium azide and produce only mono adduct even by using 1
:
2 molar ratio of terephthalonitrile
:
sodium azide (Table 2, entry 7).
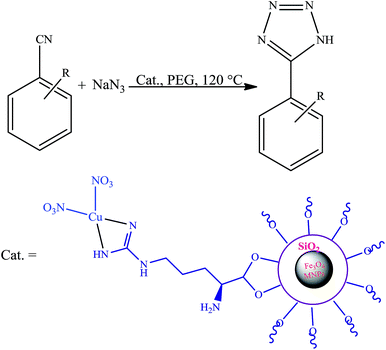 |
| Scheme 3 Synthesis of 5-substituted tetrazoles via [3 + 2] cycloaddition between nitriles and sodium azide. | |
Table 2 Synthesis of different structurally 5-substituted-1H-tetrazoles in the presence of Cu(II) immobilized on Fe3O4@SiO2@L-arginine in PEGa
Entry |
Product |
Time (min) |
Yieldb (%) |
TON |
TOF (h−1) |
Reaction conditions: aryl nitrile (1 mmol), NaN3 (1 mmol), cat. 0.030 g (1.020 mol%) in PEG (2 mL) at 120 °C. Isolated yield. |
1 |
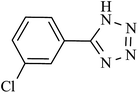 |
60 |
94 |
92.15 |
92.15 |
2 |
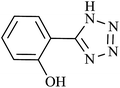 |
120 |
94 |
92.15 |
46.07 |
3 |
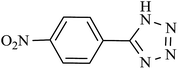 |
115 |
98 |
96.07 |
50.30 |
4 |
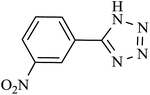 |
140 |
90 |
88.23 |
37.86 |
5 |
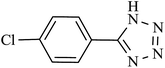 |
180 |
95 |
93.13 |
31.04 |
6 |
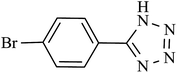 |
240 |
95 |
93.13 |
23.28 |
7 |
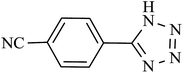 |
210 |
92 |
90.19 |
25.77 |
8 |
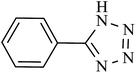 |
360 |
97 |
95.09 |
15.84 |
9 |
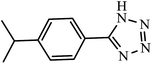 |
19 h |
98 |
96.07 |
5.05 |
10 |
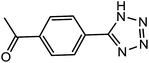 |
10 h |
68 |
66.66 |
6.66 |
11 |
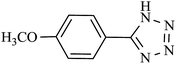 |
48 h |
60 |
58.82 |
1.22 |
The following mechanism (Scheme 4) has been suggested for described catalytic system.21 Initially, interaction of nitrogen atom of nitrile with Cu(II) forms compound I, which accelerates the cyclization step. In fact, Cu(II) nanoparticles acts as a Lewis acid and activates the nitrile group via coordination. Hence, it enhances the electrophilic character of cyanide group, which then reacts with sodium azide to form the intermediate II. Acidic work up, affords compound III and IV. The equilibrium leads to formation of the more stable tautomer IV (5-substituted-1H-tetrazole).
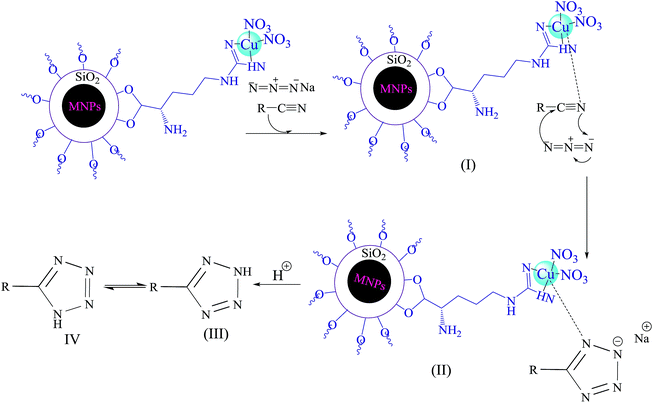 |
| Scheme 4 Proposed mechanism for Cu(II) immobilized on Fe3O4@SiO2@L-arginine catalyzed [3 + 2] cycloaddition. | |
The possibility of recovering and recycling of the catalyst is an important process from different aspects such as environmental concerns, and commercial applicable processes. In this aim, we successfully performed the recovery of catalyst from reaction mixture, which could be easily separated with an external magnet, washed three times with acetone and then with doubly distilled water several times. Then the recovered catalyst was used in the next run. This catalyst was recycled and reused at least for four times without significant loss of its catalytic activity (Fig. 5).
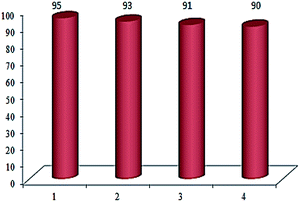 |
| Fig. 5 Reusability of the Cu(II) immobilized on Fe3O4@SiO2@L-arginine for the synthesis of 5-(4-chlorophenyl)-1H-tetrazole. | |
It seems that the supported Cu(II) leaches out from the solid support into the solvent at higher temperature and the reaction was catalyzed mainly by dissolved Cu(II). In order to consider the heterogeneity of catalyst, hot filtration technique was done for the synthesis of 5-(4-chlorophenyl)-1H-tetrazole. The catalyst separated by applying an external magnet when the reaction had proceeded to nearly 50% completion, and allowed the filtrate to react further. We found that no further reaction occurred after the separation of catalyst; this means that the Cu(II) catalyst remains on the support at elevated temperatures during the reaction.
Finally, in order to assess the present protocol with respect to other reported methods for the synthesis of 5-substituted-1H-tetrazoles; the catalytic performance of the Cu(II) immobilized on Fe3O4@SiO2@L-arginine was compared with some of the previously reported catalysts (Table 3). Eco-friendly nature and convenient recovery of the catalytic system compared to other procedures make this method as an efficient candidate for potential applications in organic synthesis.
Table 3 Comparison of various catalysts in the synthesis of 5-(4-chlorophenyl)-1H-tetrazole
Entry |
Catalyst |
Solvent |
Temperature (°C) |
Time (h) |
Yield (%)a |
Isolated yield. Chitosan supported magnetic ionic liquid nanoparticles. Cu(II) immobilized on aminated epichlorohydrin activated silica. Zn/Al hydrotalcite. |
1 |
Nano TiO2/SO4 |
DMF |
100 |
2 |
92 (ref. 22) |
2 |
CSMIL FeCl4b |
Solvent free |
70 |
7 |
84 (ref. 14) |
3 |
CAES |
DMSO |
130 |
2 |
92 (ref. 21) |
4 |
Zn/Al-HTd |
DMF |
120 |
12 |
84 (ref. 23) |
5 |
Cu-MCM-41 |
DMF |
140 |
9 |
92 (ref. 12) |
6 |
Cu(II) immobilized onc Fe3O4@SiO2@L-arginine |
PEG |
120 |
3 |
95 (this work) |
3 Conclusion
In summary, Cu(II) immobilized on Fe3O4@SiO2@L-arginine was prepared, characterized and found to be an efficient and recyclable nanocatalyst for the preparation of 5-substituted-1H-tetrazoles. To the best of our knowledge this is the first report of the synthesis of 5-substituted-1H-tetrazoles in PEG (as green solvent) instead of DMF as solvent. Other significant feature of this method is its operational simplicity, which provides an efficient method for the synthesis of different heterocyclic systems. In addition, nanocatalyst can be easily recovered by an external magnetic field and reused for subsequent run for at least 4 times with less deterioration in catalytic activity.
4 Experimental
4.1 Materials
All reagents and solvents used in this work were purchased from Sigma-Aldrich, Fluka or Merck Chemical Companies and used without further purification. The particles size and morphology were investigated by a JEOL JEM-2010 scanning electron microscopy (SEM), on an accelerating voltage of 200 kV. The thermogravimetric analysis (TGA) curves were recorded using a PL-STA 1500 device manufactured by Thermal Sciences. IR spectra were recorded as KBr pellets on a VRTEX 70 model BRUKER FT-IR spectrophotometer. Powder XRD was collected with a Rigaku-Dmax 2500 diffractometer with nickel filtered Cu Kα radiation (λ = 1.5418 Å, 40 kV).
4.2 Preparation of the Fe3O4@SiO2@L-arginine
Fe3O4 was readily synthesized by a chemical co-precipitating method similar to a previously reported work,19 followed by a SiO2-coating procedure. Briefly, 2.00 g of the obtained MNPs powder were dispersed in a mixture of 50 mL of ethanol, 20 mL of deionized water, and 10.0 mL of concentrated ammonia aqueous solution, followed by the addition of 3.36 g PEG and 2 mL of tetraethylorthosilicate (TEOS). This solution was stirred mechanically for 38 h at room temperature. Then the product, Fe3O4@SiO2, was separated by an external magnet, washed with deionized water and ethanol three times and dried at room temperature. In the second step, 1 g of Fe3O4@SiO2 was suspended in deionized water (20 mL) and sonicated until it became highly dispersed. Then, L-arginine (2 g, 0.011 mol) was added and the mixture stirred at 90 °C for 15 h. Whole synthesis was done under N2 atmosphere. The final product (Fe3O4@SiO2@L-arginine) was separated by an external magnet, washed with distilled water several times and dried in an oven overnight.
4.3 Preparation of the Cu(II) immobilized on Fe3O4@SiO2@L-arginine
In the last step, Fe3O4@SiO2@L-arginine was used as support and ligand for entrapment of Cu(II) ions. Incorporation of Cu(II) onto the Fe3O4@SiO2@L-arginine nanoparticles was carried out by mixing the Fe3O4@SiO2@L-arginine (0.5 g) and Cu(NO3)2·3H2O (0.25 g) in absolute ethanol (30 mL). The mixture was refluxed for 24 h. Finally, the synthesized nanosolid (Cu(II) immobilized on Fe3O4@SiO2@L-arginine) was separated from the suspension by magnetic decantation, washed with absolute ethanol, and dried under vacuum at room temperature (Scheme 1).
4.4 General procedure for the preparation of 5-substituted-1H-tetrazoles derivatives
A mixture of nitrile (1 mmol) and sodium azide (1 mmol) in the presence of 0.030 g of Cu(II) immobilized on Fe3O4@SiO2@L-arginine, was stirred at 120 °C in PEG; after completion of the reaction (observed on TLC), the reaction mixture was cooled down to room temperature. The catalyst was removed by magnetic field and HCl (4 N, 10 mL) added to the filtrate and corresponding tetrazole extracted with ethyl acetate (2 × 10 mL). The resultant organic layer was washed with distilled water, dried over anhydrous sodium sulfate, and concentrated to give the crude solid crystalline product.
4.5 Characterization of products
5-(3-Chlorophenyl)-1H-tetrazole (Table 2, entry 1). White solid, mp 130–134 °C (lit.:12 137), FT-IR (KBr): νmax/cm−1: 3454, 3241, 3063, 2972, 2894, 2605, 1972, 1651, 1473, 1445, 1360, 1300, 1246, 1094, 1002, 772, 675, 576. 1H NMR (400 MHz, DMSO, ppm) δ = 8.00–8.09 (m, 1H), 8.01–8.04 (m, 1H), 7.63–7.69 (m, 2H).
2-(1H-Tetrazol-5-yl)phenol (Table 2, entry 2). White solid, mp 225 °C (lit.:12 224–225), FT-IR (KBr): νmax/cm−1: 3253, 3059, 2933, 2709, 2566, 2359, 1610, 1545, 1475, 1393, 1358, 1294, 1230, 1115, 1068, 946, 808, 742, 680, 541. 1H NMR (400 MHz, DMSO, ppm) δ = 8.00–8.02 (m, 1H), 7.40–7.44 (m, 1H), 7.08–7.11 (m, 1H), 6.99–7.03 (m, 1H), 11.10 (br, 1H), 15.73 (br, 1H); 13C NMR (100 MHz, DMSO, ppm) δ = 155.7, 152, 133.1, 129.5, 120.2, 116.7, 110.9.
5-(4-Nitrophenyl)-1H-tetrazole (Table 2, entry 3). Yellow solid, mp 216–218 °C (lit.:21 218–219), FT-IR (KBr): νmax/cm−1: 3508, 3101, 2967, 2919, 2854, 2755, 2547, 1646, 1608, 1521, 1439, 1346, 1156, 1101, 1024, 951, 854, 721, 696, 494. 1H NMR (400 MHz, DMSO, ppm) δ = 8.41–8.43 (d, J = 8.8 Hz, 2H), 8.29–8.31 (d, J = 8.8 Hz, 2H).
5-(3-Nitrophenyl)-1H-tetrazole (Table 2, entry 4). White solid, mp 149–152 °C (lit.:12 154–156), FT-IR (KBr): νmax/cm−1: 3852, 3308, 3085, 2975, 2894, 2822, 2752, 1621, 1524, 1348, 1243, 1156, 1076, 1011, 912, 819, 727, 453. 1H NMR (400 MHz, DMSO, ppm) δ = 8.85 (s, 1H), 8.43–8.50 (m, 2H), 7.90–7.94 (m, 1H).
5-(4-Chlorophenyl)-1H-tetrazole (Table 2, entry 5). White solid, mp 257–260 °C (lit.:21 261–262), FT-IR (KBr): νmax/cm−1: 3436, 2769, 2634, 1908, 1607, 1484, 1432, 1265, 1155, 1091, 988, 831, 696, 505. 1H NMR (400 MHz, DMSO, ppm) δ = 8.05–8.07 (d, J = 8.4 Hz, 2H), 7.69–7.71 (d, J = 8.4 Hz, 2H).
5-(4-Bromophenyl)-1H-tetrazole (Table 2, entry 6). White solid, mp 267 °C (lit.:21 264–264), FT-IR (KBr): νmax/cm−1: 3443, 2956, 2923, 2861, 2766, 2627, 1601, 1423, 1317, 1152, 1055, 998, 842, 734, 562. 1H NMR (400 MHz, DMSO, ppm) δ = 7.97–8.00 (m, 2H), 7.81–7.84 (m, 2H).
4-(1H-Tetrazol-5-yl)benzonitrile (Table 2, entry 7). White solid, mp 191.193 °C (lit.:21 190–191), FT-IR (KBr): νmax/cm−1: 3476, 3150, 3090, 3018, 2923, 2859, 2724, 2624, 2566, 2485, 2232, 1620, 1566, 1494, 1431, 1364, 1275, 1153, 1067, 1028, 847, 749, 695, 552. 1H NMR (400 MHz, DMSO, ppm) δ = 8.21–8.24 (m, 2H), 8.09–8.12 (m, 2H); 13CNMR (100 MHz, DMSO, ppm) δ = 155.7, 133.8, 129.2, 128.1, 118.6, 113.9.
5-Phenyl-1H-tetrazole (Table 2, entry 8). White solid, mp 224–226 °C (lit.:21 214–216), FT-IR (KBr): νmax/cm−1: 3436, 2920, 1610, 1559, 1474, 1405, 1252, 1159, 1051, 987, 784, 689, 492. 1H NMR (400 MHz, DMSO, ppm) δ = 7.59–7.65 (m, 3H), 8.04–8.08 (m, 2H).
5-(4-Isopropylphenyl)-1H-tetrazole (Table 2, entry 9). White solid, mp 189 °C (lit.:24 191–192), FT-IR (KBr): νmax/cm−1: 3436, 2924, 2858, 1717, 1634, 1457, 1359, 1299, 1162, 835, 747, 541. 1H NMR (400 MHz, DMSO, ppm) δ = 7.96–7.98 (d, J = 8.4 Hz, 2H), 7.48–7.51 (d, J = 8.4 Hz, 2H), 2.94–3.04 (m, 1H), 1.25–1.26 (d, J = 6.8 Hz, 6H).
1-(4-(1H-Tetrazol-5-yl)phenyl)ethanone (Table 2, entry 10). White solid, mp 175–178 °C (lit.:25 181), FT-IR (KBr): νmax/cm−1: 3432, 3144, 3079, 3011, 2969, 2921, 2858, 2719, 2625, 2472, 1680, 1570, 1431, 1365, 1271, 1156, 1120, 1081, 1058, 997, 982, 961, 841, 752, 704, 589, 491. 1H NMR (400 MHz, DMSO, ppm) δ = 8.14–8.20 (m, 4H), 2.64 (s, 3H); 13C NMR (100 MHz, DMSO, ppm) δ = 197.9, 156.1, 138.6, 129.6, 129.5, 127.5, 27.3.
5-(4-Methoxyphenyl)-1H-tetrazole (Table 2, entry 11). White solid, mp 230–232 °C (lit.:14 230), FT-IR (KBr): νmax/cm−1: 3432, 3155, 3081, 2926, 2855, 2726, 2623, 2555, 2474, 1616, 1503, 1450, 1404, 1302, 1262, 1174, 1119, 1052, 1022, 939, 834, 804, 747, 696, 608, 519. 1H NMR (400 MHz, DMSO, ppm) δ = 7.97–8.01 (m, 2H), 7.16–7.19 (m, 2H); 3.85 (s, 3H); 13C NMR (100 MHz, DMSO, ppm) δ = 161.9, 155.1, 129.1, 116.7, 115.3, 55.9.
Acknowledgements
The authors are deeply gratitude Ilam University, Ilam, Iran for financial support of this research project.
References
- A. Sarvary and A. Maleki, Mol. Diversity, 2015, 19, 189 CrossRef CAS PubMed
. - F. Abrishami, M. Ebrahimikia and F. Rafiee, Appl. Organomet. Chem., 2015, 22, 730 CrossRef
. - M. A. E. A. Ali, A. El-Remaily and S. K. Mohamed, Tetrahedron, 2014, 70, 270 CrossRef
. - A. Palazzi, S. Stagni, S. Selva and M. Monari, J. Organomet. Chem., 2003, 669, 135 CrossRef CAS
. - H. Sharghi, S. Ebrahimpour moghaddam and M. M. Doroodmand, J. Organomet. Chem., 2013, 738, 41 CrossRef CAS
. - Z. P. Demko and K. B. Sharpless, J. Org. Chem., 2001, 66, 7945 CrossRef CAS PubMed
. - D. R. Patil, Y. B. Wagh, P. G. Ingole, K. Singh and D. S. Dalal, New J. Chem., 2013, 37, 3261 RSC
. - J. Bonnamour and C. Bolm, Chem.–Eur. J., 2009, 15, 4543 CrossRef CAS PubMed
. - G. Venkateshwarlu, A. Premalatha, K. C. Rajanna and P. K. Saiprakash, Synth. Commun., 2009, 39, 4479 CrossRef CAS
. - P. Mani, A. K. Singh and S. K. Awasthi, Tetrahedron Lett., 2014, 55, 1879 CrossRef CAS
. - M. Parveen, F. Ahmad, A. M. Malla and S. Azaz, New J. Chem., 2015, 39, 2028 RSC
. - M. Abdollahi Alibeika and A. Moaddeli, New J. Chem., 2014, 39, 2116 RSC
. - L. Bosch and J. Vilarrasa, J. Angew. Chem., 2007, 46, 3926 CrossRef CAS PubMed
. - A. Khalafi-Nezhad and S. Mohammadi, RSC Adv., 2013, 3, 4362 RSC
. - V. Polshettiwar and R. S. Varma, Tetrahedron, 2010, 66, 1091 CrossRef CAS
. - R. Mohammadi and M. Z. Kassaee, J. Mol. Catal. A: Chem., 2013, 380, 152 CrossRef CAS
. - F. Dehghani, A. R. Sardarian and M. Esmaeilpour, J. Organomet. Chem., 2013, 743, 87 CrossRef CAS
. - A. Ghorbani-Choghamarani and G. Azadi, RSC Adv., 2015, 5, 9752 RSC
. - A. Ghorbani-Choghamarani, B. Ghasemi, Z. Safari and G. Azadi, Catal. Commun., 2015, 60, 70 CrossRef CAS
. - A. Ghorbani-Choghamarani, F. Nikpour, F. Ghorbani and F. Havasi, RSC Adv., 2015, 5, 33212 RSC
. - N. Razavi and B. Akhlaghinia, RSC Adv., 2015, 5, 87769 RSC
. - M. Hosseini-Sarvari and S. Najafvand-Derikvandi, C. R. Chim., 2014, 17, 1007 CrossRef CAS
. - M. L. Kantam, K. B. Shiva Kumar and K. J. Phani Raja, J. Mol. Catal. A: Chem., 2006, 247, 186 CrossRef CAS
. - P. Sivaguru, P. Theerthagiri and A. Lalitha, Tetrahedron Lett., 2015, 56, 2203 CrossRef CAS
. - D. Amantini, R. Beleggia, F. Fringuelli, F. Pizzo and L. Vaccar, J. Org. Chem., 2004, 69, 2896 CrossRef CAS PubMed
.
|
This journal is © The Royal Society of Chemistry 2016 |
Click here to see how this site uses Cookies. View our privacy policy here.