DOI:
10.1039/D4SC04990J
(Edge Article)
Chem. Sci., 2024,
15, 18818-18824
Chiral ionic organic single-crystal and its exfoliated two-dimensional nanosheets with enhanced enantioseparation†
Received
26th July 2024
, Accepted 9th October 2024
First published on 9th October 2024
Abstract
A chiral ionic organic single-crystal (CIOC) was prepared for the first time through ionic self-assembly using bipyridium chiral ionic liquid (CIL) and 4,4′-biphenyldisulfonic acid (BDA). The CIOC can be ultrasonically exfoliated to produce two-dimensional nanosheets (2D-NSs). The 2D-NSs presented enhanced enantioseparation compared to the CIOC and CIL when used as gas chromatography stationary phase, which may be due to the exfoliated 2D-NSs exhibiting greater exposure of functional groups. Additionally, better resolution of other organic compounds such as positional isomers, n-alkanes and n-alkanols, Grob mixture, phenols and anilines was obtained in 2D-NSs than CIOC and CIL. This work not only provides a reference for the preparation of chiral ionic organic single-crystals and two-dimensional nanosheets for chiral separation, but also stimulates the preparation of such new ionic organic single-crystals via self-assembly for other potential applications.
Introduction
Chiral compounds with two configurations frequently have distinct or even diametrically opposed effects, which makes separating the racemates crucial.1–3 The similar physicochemical properties of enantiomers results in considerable challenges for their separation.4–8 Gas chromatography (GC) plays a critical role in the analysis and detection of samples9,10 due to notable advantages such as minimal sample consumption, rapid detection, and high detection sensitivity.11–13 The effectiveness of gas chromatography in separating chiral compounds mainly depends on the type and structure of the chiral stationary phase (CSP),14–17 for which cyclodextrins and their derivatives,18–20 polysaccharides and their derivatives,21–23 chiral ionic liquids (CILs),24,25 pillar[n]arene,26,27 porous organic materials,28–38etc. are widely utilized.
Of the above materials, ionic liquids possess some specific advantages such as negligible vapor pressure, high thermal stability, and strong polarity,39,40 and remain a focus for study in numerous domains at present.41,42 In 2004, CIL as stationary phase was first applied in gas chromatography by Armstrong et al.43 and then attracted much attention.44,45 Research into framework-materials based on ionic bonding has sparked our interest in ionic materials,46 however, as far as we know, there have been no reports on the preparation of chiral ionic organic single-crystals (CIOCs) and their application in chiral separation.
In this work, the synthesis of CIOC by the ionic self-assembly of a bipyridium-type CIL with 4,4′-biphenyldisulfonic acid (BDA) was proposed. It is known that 2D nanomaterials always possess the advantages of large surface area, strong interaction between host and guest, etc., which will improve the enantiomeric separation.47–49 Fittingly, this CIOC can be ultrasonically exfoliated to produce two-dimensional nanosheets (2D-NSs). Interestingly, the 2D-NSs exhibit the best performance when used as GC stationary phase for chiral separation. To the best of our knowledge, this is the first example of chiral ionic-organic single crystal material and the use of its 2D-NSs as CSP material for high-resolution gas chromatographic separation (Scheme 1).
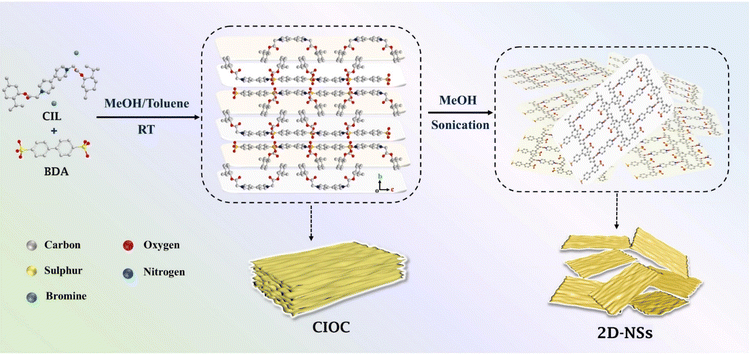 |
| Scheme 1 Synthesis of the chiral ionic organic single-crystal and two-dimensional nanosheets (viewed from the b axis). | |
Results and discussion
Preparation of CIL, CIOC and 2D-NSs
The synthesis and characterization of the CIL are shown in the ESI (Fig. S1 and S2).† Equivalent CIL and BDA were dissolved in a mixed solvent of methanol and toluene with heating, and then naturally cooled for recrystallization, and light-yellow transparent crystals were obtained as CIOC with a yield of 39.3%. Then, the CIOC was dispersed in methanol and ultrasonicated for 14 h, resulting in the formation of 2D-NSs.
Preparation of capillary columns
CIL, CIOC and 2D-NSs were coated on the capillary columns (10 m × 0.25 mm i.d.) using the sol–gel method. The detailed method can be found in the ESI.†
Characterization of CIOC and 2D-NSs
Single crystal X-ray diffraction (SXRD) provided detailed chemical composition and bonding information for the CIOC (Tables S1 and S2†), and the crystallographic data (CCDC no. 2361107) showed that the CIOC has the C2221 space group with a = 9.0954 Å, b = 228.981 Å, c = 20.898 Å, and α = β = γ = 90°. The cations and anions are intertwined with each other through the ionic bonds between the positive bipyridium N atoms on the CIL and the negative sulfamic O atoms on the BDA, and each ion is surrounded by four ions of opposite charge on the bc planes, combining in the above manner to form the monolayer (Fig. 1E). In contrast, the molecules interact with each other weakly along the a axis (Fig. 1H), and it is clear that the lamellar structure is formed by means of AB stacking, and the interlayers depend mainly on π–π interactions between the benzene rings in the anionic skeleton. Intramolecular hydrogen bonding between the O atom of the ester group and the H atom of the methyl group in the CIL also occurs within the CIOC framework, but it does not contribute to the overall structure building. It was proved that a novel ionic crystal combining 4,4′-bipyridium-based CIL and BDA was synthesized with ionic bonding as the primary mode of chemical bonding, resulting in an ordered arrangement of atoms.
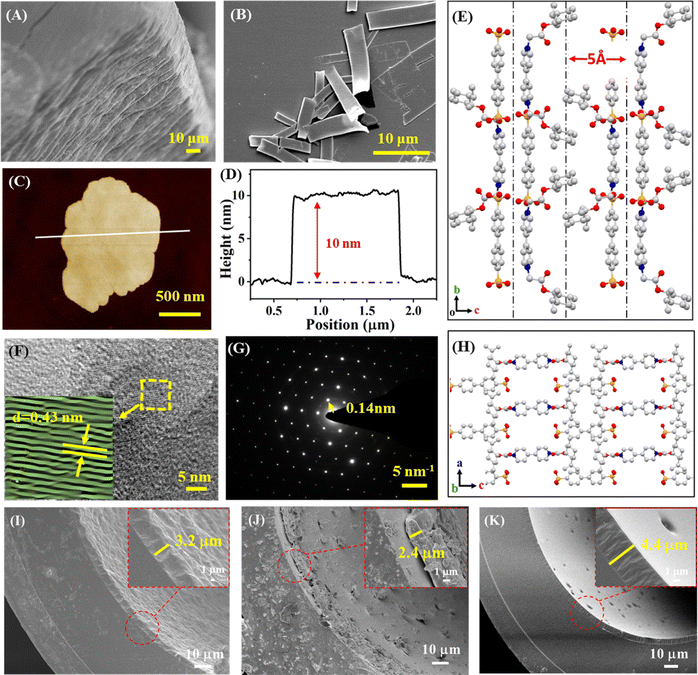 |
| Fig. 1 SEM images of the CIOC (A) and 2D-NSs (B). AFM image of the 2D-NSs (C) and related height analysis (D). Overlapping structure of the CIOC (E). HRTEM image of the 2D-NSs (F). SAED pattern of the 2D-NSs (G). The monolayer structure of the CIOC (H). SEM images showing the cross section of the CIL coated column (I), CIOC coated column (J), and 2D-NSs coated column (K). C: light grey, N: blue, O: red, S: yellow, H is omitted. | |
The scanning electron microscopy (SEM) image confirmed that the CIOC is a macro-sized lamellar stacked solid (Fig. 1A), which is consistent with the SXRD results. The Fourier transform-infrared (FT-IR) spectra (Fig. S3A†) and powder X-ray diffraction (PXRD) pattern (Fig. S3B†) prove the successful synthesis of the CIOC. 2D-NSs with repeating topological units can be obtained from CIOC via ultrasonic exfoliation. The FT-IR spectrum and XRD pattern of the 2D-NSs confirmed that the composition and crystal structure remain unchanged (Fig. S3†). The SEM image (Fig. 1B) showed that the exfoliated CIOC was lamellar. The atomic force microscopy (AFM) image revealed lamellae with sharp edges (Fig. 1C), and the height was measured to be about 10 nm (Fig. 1D), which proved that 2D-NSs were successfully prepared. The CIOC is a 2D crystal formed by π–π stacking between different layers, with a distance of approximately 0.5 nm between layers (Fig. 1E). It can be inferred that the 2D-NSs obtained through exfoliation consist of approximately 20 monolayers. High-resolution transmission electron microscopy (HRTEM) revealed well-ordered linear features with a lattice spacing of 0.43 nm (Fig. 1F), demonstrating that the 2D-NSs still maintain good crystalline structure. The selected area electron diffraction (SAED) pattern of the 2D-NSs revealed nearest reflections at 0.14 nm (Fig. 1G), confirming the existence of the crystalline structure of the exfoliated 2D-NSs with regular diffraction points. Fig. S3C† confirms that both the CIL and 2D-NSs are optically active and also reveals the chiral retention of the synthesized nanosheets. The CIL appears as three signal peaks on the circular dichroism (CD) spectrum with a g-factor of about 4 × 10−4 (Fig. S3D†). In contrast, both CD signal peaks of the 2D-NSs show a red-shift (Fig. S3E†), and the g-factor was also reduced, which might originate from the effect of the BDA.
The N2 adsorption experiment (Fig. S3F†) demonstrated that both the CIOC and 2D-NSs have a type II isotherm, and the Brunauer–Emmett–Teller (BET) surface area of the 2D-NSs is significantly increased, which suggests that while both the CIOC and 2D-NSs are non-porous structures, the specific surface area is enhanced by the 2D-NSs produced during exfoliation. Compared to bulk CIOCs, the 2D-NSs expose more interaction sites and have stronger interaction forces with analytes, resulting in a superior selective separation, confirmed by the results of the N2 adsorption experiment.50 Thermogravimetric analysis (TGA) showed that the CIL remained stable until the temperature reached 277 °C (Fig. S4A†). In addition, the CIOC and 2D-NSs are thermally stable with the CIL (Fig. S4B and C†); all of them possessed good thermal stability.
Characterizations of capillary columns
SEM images were utilized to show the morphology of the inner wall of the capillary columns. Fig. 1I indicates the successful deposition of the CIL on the inner wall of the capillary with a thickness of 3.2 μm. In Fig. 1J, the inner wall of the capillary column shows the CIOC coating, which has a 2.4 μm coating thickness and takes the shape of lumps with variable sizes. Fig. 1K reveals the presence of protrusions on the surface of the gel layer with sizes of 4.4 μm, confirming the successful preparation of the 2D-NSs coated capillary columns. Fig. S4D† shows that the three proposed columns retain relatively good column stability.
Five probe molecules, including benzene (X′), n-butanol (Y′), 2-pentanone (Z′), 1-nitropropane (U′), and pyridine (S′) were used to measure the polarity of the stationary phase using a polarity index.51,52 The results for the three coated columns showed that they exhibited moderate polarity and were comparable to one another (Table S3†).
Separation of chiral compounds
The separation of chiral compounds has always been a challenging task. In Fig. 2, one pair of cis-/trans-isomers (decahydronaphthalene) and 3 enantiomers, mandelonitrile, epichlorohydrin and benzoin, were separated as test compounds. For decahydronaphthalene and mandelonitrile, the resolution (Rs) and selective factor (α) of the 2D-NSs coated capillary column reached 14.85 and 4.86, and the values for the CIOC coated columns reached 11.00 and 2.99, whereas the values for the CIL coated column were 6.85 and 2.64. The enantiomer separation ability can be reasonably determined to be in the following order: 2D-NSs > CIOC > CIL based on the separation results. This improvement can be attributed to the connection between the CIL and BDA increasing hydrogen bonding, dipole–dipole, and π–π interactions, as well as the introduction of certain steric hindrance effects. Regarding benzoin, the 2D-NSs coated column was able to achieve the baseline separation, whereas the CIOC coated column showed no signs of separation. Compared to bulk CIOC, the 2D-NSs exposed more interaction sites and exhibited stronger interaction forces with analytes, resulting in superior selective separation, confirmed by the results of the N2 adsorption experiment. Commercialized β-DEX 120 was used to make comparisons, with results such as those in Table S7,† where 2D-NSs coated capillary columns still exhibited enantiomeric separation advantages.
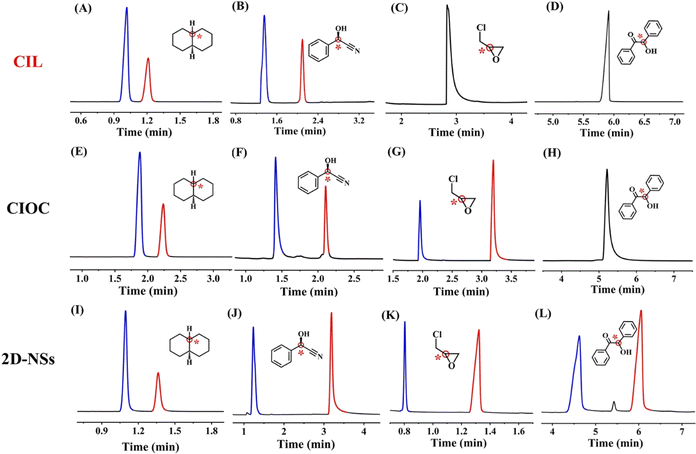 |
| Fig. 2 Representative gas chromatograms for the separation of cis-/trans-isomers and enantiomers on CIL-/CIOC/2D-NSs-coated columns: decahydronaphthalene (A, E, and I); mandelonitrile (B, F, and J); epichlorohydrin (C, G, and K); and benzoin (D, H, and L). Separation conditions are shown in Tables S4–6.† Derivatization can be found in the ESI.† | |
In order to investigate the thermodynamic parameters of the separation on CIL, CIOC and 2D-NSs coated columns, we separated the cis-/trans-decahydronaphthalene isomers at different temperatures (Fig. S5A–C†). The reduction in retention time and Rs as the temperature increases indicates an exothermic separation process for cis-/trans-decahydronaphthalene. The relevant Van't Hoff equations (Fig. S5D–F†) showed excellent linearity, confirming that the separation mechanism of cis-/trans-decahydronaphthalene was not altered. Negative values of the Gibbs free energy change (ΔG) suggest the spontaneity of the separation process, while negative entropy change (ΔS) and enthalpy change (ΔH) values collectively govern the separation process throughout, as shown in Table S8.†
To further elucidate the mechanism of separation and explore the microscopic interaction pattern between chiral compounds and the stationary phase, energy-minimized DFT calculations and relevant NCI studies have been carried out for epichlorohydrin molecules with the CIL or CIOC. As shown in Fig. 3A and B, (R/S)-epichlorohydrin molecules (oxygen and hydrogen atoms) have multiple hydrogen bonding interactions with CIOC (hydrogen and oxygen atoms on the cation and oxygen atoms on the anion), with bond lengths ranging from 2.28–3.05 Å. The binding energy of CIOC@(S)-epichlorohydrin was more negative than that of (R)-epichlorohydrin, which proved that the interactions between (R)-epichlorohydrin and CIOC were stronger, and thus the CIOC had stronger retention of (S)-epichlorohydrin, in agreement with the experimental results. Epichlorohydrin could not be separated on the CIL coated column on account of the two conformations of the molecules being combined with CIL through hydrogen bonding with little difference in binding energies and bond distances between 2.62 and 3.00 Å (Fig. 3C and D). The calculations mentioned above proved that the CIOC structure is more favorable for enantiomeric separation since the inclusion of BDA provides additional hydrogen-bonding interactions and increases the difference in binding energies of chiral compounds of different configurations.
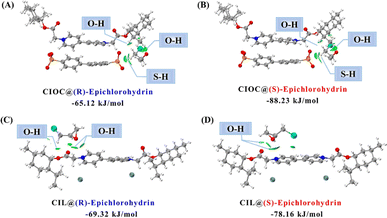 |
| Fig. 3 Energy-minimized DFT and NCI analysis for CIOC@(R)-epichlorohydrin (A), CIOC@(S)-epichlorohydrin (B), CIL@(R)-epichlorohydrin (C), and CIL@(S)-epichlorohydrin (D). | |
Separation of achiral compounds
Positional isomers, serving as crucial intermediates in various fields, play an essential function in production and biology.53,54 Good separation of the three positional isomers of nitroaniline, nitrotoluene, and dimethylphenol is shown in Fig. 4, and related chromatographic data are shown in Table S9.† The results indicate that the coated columns exhibit separation capabilities for positional isomers that are comparable to the commercial AB-5MS column (Fig. S6†).
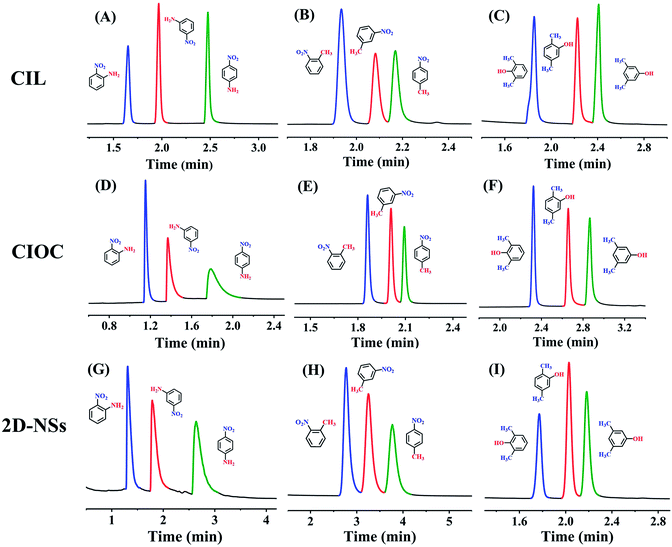 |
| Fig. 4 Gas chromatograms of positional isomers on CIL, CIOC and 2D-NSs coated capillary columns: nitroaniline (A, D, and G), nitrotoluene (B, E, and H), and dimethylphenol (C, F, and I). Oven program: 100–170 °C at 30 °C min−1 for (A), 50–150 °C at 30 °C min−1 for (B), 70–150 °C at 20 °C min−1 for (C), 120–180 °C at 50 °C min−1 for (D), 80–140 °C at 30 °C min−1 for (E), 100–150 °C at 20 °C min−1 for (F, and G), 90–160 °C at 20 °C min−1 for (H), and 120–150 °C at 20 °C min−1 for (I). Flow rate: 3.0 mL min−1 for (A, and B); 2.0 mL min−1 for (C, E, and G); 4.0 mL min−1 for (D); 1.5 mL min−1 for (F); 0.8 mL min−1 for (H); and 1.0 mL min−1 for (I). | |
These constructed chiral columns not only performed well for chiral and isomer separation, but also performed well in the separation of other non-polar to polar compounds, including n-alkanes, n-alkanols, Grob mixture, phenols, anilines and benzene derivatives, with the 2D-NSs coated column exhibiting the best separation performance (chromatograms, related chromatographic conditions, Rs and α are shown in Fig. S7–S9 and Table S10†). By investigating the repeatability and stability of the CIL, CIOC and 2D-NSs coated capillary columns using xylenol isomers as models (Fig. S10, and Table S11†), it was found that the 2D-NSs coated column exhibited good results.
Conclusions
In conclusion, a novel chiral ionic organic single-crystal material was rationally designed and creatively prepared. It was formed by self-assembling recrystallization between organic anions and cations. Furthermore, the corresponding 2D-NSs were constructed through ultrasonic exfoliation. Excellent recognition ability and separation selectivity for chiral compounds was obtained using the 2D-NSs coated capillary column, which is due to the presence of more exposed functional interaction sites. In addition, this novel ionic organic single-crystal and 2D-NSs coated capillary column exhibited good separation of positional isomers, Grob mixture, alkylbenzenes, etc. This discovery offers a crucial route for the synthesis of novel chiral ionic organic single-crystals via ionic self-assembly and related 2D nanomaterials for chiral separation and other potential applications.
Data availability
All the relevant data of this study are available within the manuscript and its ESI.†
Author contributions
Huifeng Liu: experiment, data curation, visualization, investigation, writing – original draft, validation. Yongrui He: investigation, validation. Jia Chen: conceptualization, discussion, supervision, project administration, funding acquisition, writing – review & editing, validation. Xiaoqing Qu: visualization, validation. Jing He: visualization, validation. Xuwei Chen: supervision, validation. Jianhua Wang: visualization, validation. Hongdeng Qiu: conceptualization, supervision, discussion, writing – review & editing, validation. All authors have given approval to the final version of the manuscript.
Conflicts of interest
There are no conflicts to declare.
Acknowledgements
This work was supported by the National Natural Science Foundation of China (22374159), Gansu Province Outstanding youth Foundation (24JRRA042), the “Light of West China” Program from Chinese Academy of Science (xbzg-zdsys-202008), the Youth Innovation Promotion Association CAS (2021420) and Natural Science Foundation of Shandong Province ZR2022QB246. We also want to express our gratitude to Dr Chen Yuan from Sichuan University for his good suggestion.
Notes and references
- T. Chen, H. Li, X. Shi, J. Imbrogno and D. Zhao, J. Am. Chem. Soc., 2024, 146, 14433–14438 CrossRef CAS PubMed.
- N. Xu, K. Su, E.-S. M. El-Sayed, Z. Ju and D. Yuan, Chem. Sci., 2022, 13, 3582–3588 RSC.
- X. Kang, E. R. Stephens, B. M. Spector-Watts, Z. Li, Y. Liu, L. Liu and Y. Cui, Chem. Sci., 2022, 13, 9811–9832 RSC.
- H. Li, X. Wang, C. Shi, L. Zhao, Z. Li and H. Qiu, Chin. Chem. Lett., 2023, 34, 107606 CrossRef CAS.
- C. Zhu, K. Yang, H. Wang, Y. Fang, L. Feng, J. Zhang, Z. Xiao, X. Wu, Y. Li, Y. Fu, W. Zhang, K. Wang and H. Zhou, ACS Cent. Sci., 2022, 8, 562–570 CrossRef CAS PubMed.
- Y. Huang, H. Zeng, L. Xie, R. Gao, S. Zhou, Q. Liang, X. Zhang, K. Liang, L. Jiang and B. Kong, J. Am. Chem. Soc., 2022, 144, 13794–13805 CrossRef CAS PubMed.
- S. Zhang, J. Zhou and H. Li, Angew. Chem., Int. Ed., 2022, 61, e202204012 CrossRef CAS PubMed.
- T. Miyabe, H. Iida, A. Ohnishi and E. Yashima, Chem. Sci., 2012, 3, 863–867 RSC.
- Y. Cui, C. Yang and X. Yan, ACS Appl. Mater. Interfaces, 2020, 12, 4954–4961 CrossRef CAS PubMed.
- W. Kou, C. Yang and X. Yan, J. Mater. Chem. A, 2018, 6, 17861–17866 RSC.
- Q. Song, J. Yang, K. Zheng, T. Zhang, C. Yuan, L. Yuan and X. Hou, J. Am. Chem. Soc., 2024, 146, 7594–7604 CrossRef CAS PubMed.
- M. Xu, P. Cai, S. Meng, Y. Yang, D. Zheng, Q. Zhang, L. Gu, H. Zhou and Z. Gu, Angew. Chem., Int. Ed., 2022, 61, e202207786 CrossRef CAS PubMed.
- J. Chen, Y. Huang, X. Wei, X. Lei, L. Zhao, M. Guan and H. Qiu, Chem. Commun., 2019, 55, 10908–10911 RSC.
- M. N. Corella-Ochoa, J. B. Tapia, H. N. Rubin, V. Lillo, J. González-Cobos, J. L. Núñez-Rico, S. R. G. Balestra, N. Almora-Barrios, M. Lledós, A. Güell-Bara, J. Cabezas-Giménez, E. C. Escudero-Adán, A. Vidal-Ferran, S. Calero, M. Reynolds, C. Martí-Gastaldo and J. R. Galán-Mascarós, J. Am. Chem. Soc., 2019, 141, 14306–14316 CrossRef CAS PubMed.
- H. Deng, X. Wu, L. Zhang, J. Shen, Y. Qiao, X. Wang, C. Bai, T. Zheng and Y. Okamoto, Carbohydr. Polym., 2022, 296, 119888 CrossRef CAS PubMed.
- C. Yuan, W. Jia, Z. Yu, Y. Li, M. Zi, L. Yuan and Y. Cui, J. Am. Chem. Soc., 2022, 144, 891–900 CrossRef CAS PubMed.
- H. Qian, C. Yang and X. Yan, Nat. Commun., 2016, 7, 12104 CrossRef CAS PubMed.
- Y. Wang, H. Chen, Y. Xiao, C. H. Ng, T. S. Oh, T. T. Y. Tan and S. C. Ng, Nat. Protoc., 2011, 6, 935–942 CrossRef CAS PubMed.
- B. Tang, W. Wang, H. Hou, Y. Liu, Z. Liu, L. Geng, L. Sun and A. Luo, Chin. Chem. Lett., 2022, 33, 898–902 CrossRef CAS.
- S. Lv, C. Ma, H. Cong, Y. Shen and B. Yu, Sep. Purif. Technol., 2022, 294, 121147 CrossRef CAS.
- B. Chankvetadze, Trends Anal. Chem., 2020, 122, 115709 CrossRef CAS.
- X. Wang, H. Li, K. Quan, L. Zhao, H. Qiu and Z. Li, Talanta, 2021, 225, 121987 CrossRef CAS PubMed.
- T. Kubota, C. Yamamoto and Y. Okamoto, J. Am. Chem. Soc., 2000, 122, 4056–4059 CrossRef CAS.
- L. Dong, J. Xia, H. Song, J. Yang, Y. Sun, X. Li and X. Zhu, J. Mol. Liq., 2023, 371, 120982 CrossRef CAS.
- T. Sun, M. Ba, Y. Song, W. Li, Y. Zhang, Z. Cai, S. Hu, X. Liu, D. Nardiello and M. Quinto, Anal. Chim. Acta, 2024, 1291, 342221 CrossRef CAS PubMed.
- C. Shi, H. Li, X. Shi, L. Zhao and H. Qiu, Chem. Commun., 2022, 58, 3362–3365 RSC.
- Y. Wu, M. Tang, Z. Wang, L. Shi, Z. Xiong, Z. Chen, J. L. Sessler and F. Huang, Nat. Commun., 2023, 14, 4927 CrossRef CAS PubMed.
- C. Liu, K. Quan, H. Li, X. Shi, J. Chen and H. Qiu, Chem. Commun., 2022, 58, 13111–13114 RSC.
- C. Yuan, Z. Wang, W. Xiong, Z. Huang, Y. Lai, S. Fu, J. Dong, A. Duan, X. Hou, L. Yuan and Y. Cui, J. Am. Chem. Soc., 2023, 145, 18956–18967 CrossRef CAS PubMed.
- N. Huang, L. Zhai, D. E. Coupry, M. A. Addicoat, K. Okushita, K. Nishimura, T. Heine and D. Jiang, Nat. Commun., 2016, 7, 12325 CrossRef PubMed.
- A. A. Kotova, D. Thiebaut, J. Vial, A. Tissot and C. Serre, Coord. Chem. Rev., 2022, 455, 214364 CrossRef CAS.
- Y. Wang, J. Chen, L. Xiong, B. Wang, S. Xie, J. Zhang and L. Yuan, Anal. Chem., 2022, 94, 4961–4969 CrossRef CAS PubMed.
- S. Xie, X. Chen, J. Zhang and L. Yuan, Trends Anal. Chem., 2020, 124, 115808 CrossRef CAS.
- X. Han, J. Huang, C. Yuan, Y. Liu and Y. Cui, J. Am. Chem. Soc., 2018, 140, 892–895 CrossRef CAS PubMed.
- J. Zhang, S. Xie, L. Chen, B. Wang, P. He and L. Yuan, Anal. Chem., 2015, 87, 7817–7824 CrossRef CAS PubMed.
- A. Kewley, A. Stephenson, L. Chen, M. E. Briggs, T. Hasell and A. I. Cooper, Chem. Mater., 2015, 27, 3207–3210 CrossRef CAS.
- J. Xiao, J. Chen, J. Liu, H. Ihara and H. Qiu, Green Energy Environ., 2023, 8, 1596–1618 CrossRef CAS.
- J. Chen, Y. Wang, Y. Yu, J. Wang, J. Liu, H. Ihara and H. Qiu, Exploration, 2023, 3, 20220144 CrossRef PubMed.
- K. Dong, X. Liu, H. Dong, X. Zhang and S. Zhang, Chem. Rev., 2017, 117, 6636–6695 CrossRef CAS PubMed.
- M. Costa Gomes, L. Pison, C. Červinka and A. Padua, Angew. Chem., Int. Ed., 2018, 57, 11909 CrossRef CAS PubMed.
- Q. Zhao, M. Yin, A. P. Zhang, S. Prescher, M. Antonietti and J. Yuan, J. Am. Chem. Soc., 2013, 135, 5549–5552 CrossRef CAS PubMed.
- J. Sun, H. Lin, W. Zhang, M. Gao, M. Antonietti and J. Yuan, Mater. Horiz., 2017, 4, 681–687 RSC.
- J. Ding, T. Welton and D. W. Armstrong, Anal. Chem., 2004, 76, 6819–6822 CrossRef CAS PubMed.
- H. Liu, J. Chen, M. Chen, J. Wang and H. Qiu, Anal. Chim. Acta, 2023, 1274, 341496 CrossRef CAS PubMed.
- H. Liu, P. Jin, F. Zhu, L. Nie and H. Qiu, Trends Anal. Chem., 2021, 134, 116132 CrossRef CAS.
- M. O'Shaughnessy, J. Glover, R. Hafizi, M. Barhi, R. Clowes, S. Y. Chong, S. P. Argent, G. M. Day and A. I. Cooper, Nature, 2024, 630, 102–108 CrossRef PubMed.
- M. Xu, P. Cai, S. S. Meng, Y. Yang, D. S. Zheng, Q. H. Zhang, L. Gu, H. C. Zhou and Z. Y. Gu, Angew. Chem., Int. Ed., 2022, 61, e202207786 CrossRef CAS PubMed.
- C. Tan, K. Yang, J. Dong, Y. Liu, Y. Liu, J. Jiang and Y. Cui, J. Am. Chem. Soc., 2019, 141, 17685–17695 CrossRef CAS PubMed.
- J. Dong, L. Liu, C. Tan, Q. Xu, J. Zhang, Z. Qiao, D. Chu, Y. Liu, Q. Zhang, J. Jiang, Y. Han, A. P. Davis and Y. Cui, Nature, 2022, 602, 606–611 CrossRef CAS PubMed.
- J. Guo, Y. Zhang, Y. Zhu, C. Long, M. Zhao, M. He, X. Zhang, J. Lv, B. Han and Z. Tang, Angew. Chem., Int. Ed., 2018, 57, 6873–6877 CrossRef CAS PubMed.
- T. Ma, W. Deng, H. Qian, S. Xu, C. Yang and X. Yan, ACS Mater. Lett., 2024, 6, 1616–1622 CrossRef CAS.
- Z. Wang, C. Yang and X. Yan, J. Chromatogr. A, 2019, 1608, 460420 CrossRef CAS PubMed.
- C. Shi, H. Li, X. Shi, L. Zhao and H. Qiu, Chin. Chem. Lett., 2022, 33, 3613–3622 CrossRef CAS.
- B. Huang, K. Li, Q. Ma, T. Xiang, R. Liang, Y. Gong, B. Wang, J. Zhang, S. Xie and L. Yuan, Anal. Chem., 2023, 95, 13289–13296 CrossRef CAS PubMed.
|
This journal is © The Royal Society of Chemistry 2024 |
Click here to see how this site uses Cookies. View our privacy policy here.