Heterogeneous biomimetic aerobic synthesis of 3-iodoimidazo[1,2-a]pyridines via CuOx/OMS-2-catalyzed tandem cyclization/iodination and their late-stage functionalization†
Received
14th July 2014
, Accepted 18th August 2014
First published on 20th August 2014
Abstract
In the presence of copper supported on manganese oxide-based octahedral molecular sieves OMS-2 (CuOx/OMS-2), the heterogeneously catalytic, aerobic synthesis of 3-iodoimidazo[1,2-a]pyridines from acetophenones, 2-aminopyridines and I2via tandem cyclization/iodination in a one-pot manner is achieved. As a heterogeneous catalyst, OMS-2 acts not only as a support for catalytic Cu species but also as an electron-transfer mediator (ETM), which combines with Cu to generate a low-energy pathway for rapid electron transfer. In this way, the biomimetic, catalytic oxidation could directly employ air as a green terminal oxidant under mild conditions, and provide corresponding products with broad substrates in moderate to excellent yields using very low catalyst loading (0.2 mol% Cu). In this process, I2 not only plays the role of catalyst for the initial cyclization, with assistance from CuOx/OMS-2, but also acts as a reactant for the next electrophilic oxidative iodination, which makes the reaction highly atom economic. Besides, the late-stage functionalization of the I-substituted imidazo[1,2-a]pyridines is also demonstrated by various coupling reactions, which show its potential applications in synthetic and pharmaceutical chemistry. Moreover, the catalyst is truly heterogeneous and reusable.
Introduction
With the increase of molecular complexity, one-pot multi-step reactions are gradually becoming superior and attractive because they reduce the number of workups and purifications required. Therefore, employing one-pot tandem reactions can avoid stop-and-go synthesis and the subsequent cost for waste disposal, which means it is beneficial to the economy and the environment.1 Furthermore, it is more meaningful and profitable to apply one-pot tandem oxidation reactions in heterogeneous catalysis using air as a green oxidant since heterogeneous catalysis has practical advantages in catalyst handling, recyclability and separation of the catalyst from the products.2–4 Consequently, the development of heterogeneously catalytic, aerobic one-pot tandem oxidation reactions is highly desirable.
Imidazo[1,2-a]pyridines are significant pharmacophores and are widely found in many biologically active compounds. They have antibacterial,5 antiviral,6 antitumor7 and anti-inflammatory8 properties, thus they are present in many best-selling drugs, such as zolpidem, alpidem, necopidem, saripidem and zolimidine.9 A variety of synthetic methodologies have been developed,10 focusing on (1) condensation between 2-aminopyridines and precursors like α-haloketones;11 (2) Ag-mediated cyclization of 2-aminopyridines and alkynes;12 (3) Fe-catalyzed cyclization of 2-aminopyridines and nitroolefins,13 and recently, (4) Cu-catalyzed cyclization of 2-aminopyridines and ketones with the assistance of I−.14 In terms of heterogeneous catalysis, we have recently reported a solid-supported CuCl2/nano TiO2-catalyzed oxidative cyclization of ketones and 2-aminopyridines without the assistance of I−.15 Although exciting results have been discovered, the synthesis of halo-substituted imidazo[1,2-a]pyridines is rarely reported because the halide cannot be maintained on the 3-position of imidazo[1,2-a]pyridines as long as α-haloketones are employed for condensation with 2-aminopyridines. Recently, Jiang's group developed a homogeneous Cu(OTf)2-catalyzed method for the synthesis of 2-halo-substituted imidazo[1,2-a]pyridines using 2-aminopyridines and haloalkynes (Scheme 1a).16 To develop economic and environmentally benign catalytic reactions, heterogeneous catalysis for the synthesis of halo-substituted imidazo[1,2-a]pyridines is required.
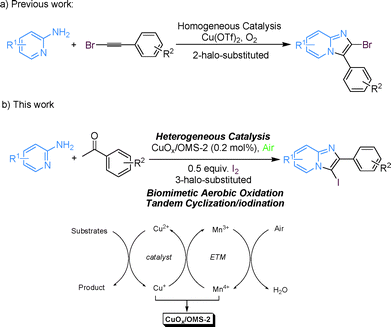 |
| Scheme 1 (a) Homogeneous synthesis of 2-halo-substituted imidazo[1,2-a]pyridines. (b) Heterogeneous biomimetic oxidative synthesis of 3-iodoimidazo[1,2-a]pyridines. | |
In order to use oxygen or hydrogen peroxide directly as oxidants in oxidation reactions, Bäckvall and co-workers developed a biomimetic oxidative methodology that facilitated the procedures by employing electron-transfer mediators (ETMs) to decrease the redox energy barrier between the catalyst and the oxidant (Scheme 2).17 Under this circumstance, ETMs generate a low-energy pathway for rapid electron transfer, which usually increases the efficiency (decreases the catalyst loading), thus complementing direct oxidation reactions.18 Based on the biomimetic oxidative concept, Mizuno's group designed a copper hydroxide catalyst supported on a manganese oxide-based octahedral molecular sieve OMS-2 (Cu(OH)x/OMS-2), which facilitated the oxidative homo-coupling of alkynes by the generation of a rapid electron-transfer path.19 Octahedral molecular sieve (OMS) materials composed of manganese oxide are crystalline, porous, mixed valent and semi-conductive; therefore they are applied in catalysis, battery materials, ion-exchange and adsorption.20–22 We noticed that the redox potential of the MnO2/Mn2O3 reaction is 1.04 V, which is between the potentials of CuO/Cu2O (0.75 V) and O2/H2O (1.23 V).4a,e,23 Thus it is possible to prepare the heterogeneous catalyst CuOx/OMS-2 and perform oxidation reactions using it to decrease the redox energy barrier. In the course of investigating the applications of heterogeneous catalysts in our group,24 we proposed that copper acts as a substrate-selective redox catalyst while OMS-2 behaves as a combination of an ETM and a support, thereby forming a low-energy pathway to make electrons transfer rapidly in the heterogeneous oxidative process. In the oxidative process, Cu oxidizes the substrates, then reduced Cu is reoxidized by Mn and reduced Mn is subsequently reoxidized by O2 (Scheme 1b).
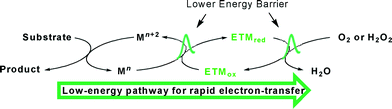 |
| Scheme 2 Biomimetic catalytic oxidation in the presence of ETMs. | |
Herein, we describe the heterogeneous biomimetic aerobic synthesis of 3-iodoimidazo[1,2-a]pyridines via CuOx/OMS-2-catalyzed tandem cyclization/iodination from 2-aminopyridines, ketones and I2 (Scheme 1b). In the oxidative procedure, OMS-2 works as a support with a large surface and an ETM that facilitates rapid electron transfer by means of decreasing the energy barrier between the catalyst and the oxidant, while I2 is used as a catalyst for the initial cyclization as well as dedicating itself entirely to the subsequent electrophilic oxidative iodination.
Results and discussion
The one-pot tandem oxidative synthesis of 3-iodoimidazo[1,2-a]pyridine from 2-aminopyridine, acetophenone and I2 was studied using different catalysts and solvents to optimize the reaction conditions (Table 1). We anticipated the tandem reaction to go through the I-catalyzed Ortoleva–King reaction14e,15 with the assistance of copper to form imidazo[1,2-a]pyridine followed by the copper-catalyzed iodination of imidazo[1,2-a]pyridine, which indicates that the high yield of imidazo[1,2-a]pyridine is necessary to form the I-substituted product. As expected, imidazo[1,2-a]pyridine, 3aa, was the major product if the reaction was run without adding the metal catalyst (Table 1, entry 1). When OMS-2 was directly used as the catalyst, a moderate yield of the desired product, 3a, was obtained although 3aa was still isolated at 29% yield (Table 1, entry 2). To our delight, supported Cu(OH)x/OMS-2 and CuOx/OMS-2 (6 mg, 0.2 mol%, Cu: 1.3 wt%; see the Experimental section for details of the preparation) brought about good results, and the latter one provided only the desired 3-iodoimidazo[1,2-a]pyridine in excellent yield (Table 1, entries 3 and 4). Subsequently, unsupported CuO was not found to be efficient for this tandem reaction, even though a longer reaction time was employed (Table 1, entry 5). In addition, a physical mixture of bulk CuO (1 mol%) and OMS-2 (6 mg) did not selectively give 3a in high yield (Table 1, entry 6). These results demonstrated that highly dispersed copper species on OMS-2 could play a key role during the tandem reaction. So CuOx/OMS-2 was chosen to optimize the reaction temperature. It was found that temperatures below 100 °C could not offer the desired product at all and that the reaction stopped at the initial cyclization rather than finishing the next iodination (Table 1, entries 7–9). However, a higher temperature (150 °C) did not result in a higher yield, which means that the heterogeneous catalyst allowed the reaction to be carried out at a mild temperature (Table 1, entry 10). Then, various solvents were studied in the reaction and only polar DCB (o-dichlorobenzene) could provide the desired product while the other examined solvents just offered the cyclized product to some extent (Table 1, entries 11–14). Notably, DMSO led to 2-aryl-3-(pyridine-2-ylamino)imidazo[1,2-a]pyridine as the only product instead of 3a and 3aa (Table 1, entry 11), which was the same as the discovery by Wu's group.25 Regarding the amount of catalyst, inferior results were found if the loading of CuOx/OMS-2 or the amount of Cu supported on OMS-2 were decreased (Table 1, entries 15 and 16). On the other hand, an increased loading of the heterogeneous catalyst likewise could not increase the yield (Table 1, entry 17). Subsequently, it was found that the atmosphere of the reaction seriously affected the tandem reaction (Table 1, entries 18 and 19). N2 suppressed the iodination of the tandem reaction completely while O2 was favorable to it, which means that the reaction ended at the first stage (cyclization) without the oxidant and that the iodination of that tandem reaction is aerobic. Thus, air was chosen to be an efficient green oxidant for this tandem reaction. Moreover, an extended reaction time also did not increase the yield of the iodo-substituted product (Table 1, entry 20). Finally, the controlled experiment under iodine-free conditions did not give any products at all, which confirmed that the tandem reaction mechanically undergoes an initial I-catalyzed Ortoleva–King reaction with the assistance of copper (Table 1, entry 21).
Table 1 Optimization of the reaction conditionsa

|
Entry |
Catalyst |
Solvent |
Temp. (°C) |
Yieldb (%) |
3a
|
3aa
|
Reaction conditions: 2-aminopyridine (0.6 mmol), acetophenone (0.5 mmol), I2 (0. 25 mmol), catalyst (6 mg), solvent (1 mL), air, 20 h.
Isolated yields.
For 48 h.
6 mg of CuOx/OMS-2 (Cu: 0.3 wt%) was used.
3 mg of CuOx/OMS-2 was used.
12 mg of CuOx/OMS-2 was used.
N2 atmosphere.
O2 atmosphere.
In the absence of I2.
|
1 |
|
DCB |
100 |
10 |
89 |
2 |
OMS-2 |
DCB |
100 |
50 |
29 |
3 |
Cu(OH)x/OMS-2 |
DCB |
100 |
81 |
0 |
4 |
CuOx/OMS-2 |
DCB |
100 |
93 |
0 |
5 |
CuO |
DCB |
100 |
8 (14c) |
75 (60c) |
6 |
CuO + OMS-2 |
DCB |
100 |
52 |
30 |
7 |
CuOx/OMS-2 |
DCB |
r.t. |
0 |
39 |
8 |
CuOx/OMS-2 |
DCB |
50 |
0 |
45 |
9 |
CuOx/OMS-2 |
DCB |
80 |
0 |
63 |
10 |
CuOx/OMS-2 |
DCB |
150 |
89 |
0 |
11 |
CuOx/OMS-2 |
DMSO |
100 |
0 |
0 |
12 |
CuOx/OMS-2 |
DMF |
100 |
0 |
20 |
13 |
CuOx/OMS-2 |
o-Xylene |
100 |
0 |
32 |
14 |
CuOx/OMS-2 |
1,4-Dioxane |
100 |
0 |
45 |
15d |
CuOx/OMS-2 |
DCB |
100 |
48 |
0 |
16e |
CuOx/OMS-2 |
DCB |
100 |
72 |
0 |
17f |
CuOx/OMS-2 |
DCB |
100 |
90 |
0 |
18g |
CuOx/OMS-2 |
DCB |
100 |
0 |
78 |
19h |
CuOx/OMS-2 |
DCB |
100 |
92 |
0 |
20c |
CuOx/OMS-2 |
DCB |
100 |
85 |
0 |
21i |
CuOx/OMS-2 |
DCB |
100 |
0 |
0 |
Next, more tests using different heterogeneous catalysts were carried out for further optimization of the reaction conditions (Table 2). Firstly, many bimetallic catalysts loaded on various supports were applied in the tandem reaction. CuOx–MnOx/Al–Ti did not lead to an improvement in the overall efficiency, although Cu and Mn were supposed to generate a low-energy path as CuOx/OMS-2 does (Table 2, entries 1 and 2). In addition, other bimetallic catalysts, like CuOx–FeOx/OMS-2 and CuOx–FeOx/C, likewise did not improve the tandem reaction (Table 2, entries 3 and 4). Lastly, the reactions were performed using copper oxide loaded on different supports as heterogeneous catalysts (Table 2, entries 5–8). Consequently, Al–Ti, nano-TiO2, charcoal and ATP (attapulgite) did not show better results than OMS-2, though CuOx/nano-TiO2 provided the desired product in moderate yield. Therefore, it was proven that OMS-2 is the most suitable support for the reaction (Table 2, entries 1 and 5–8).
Table 2 Optimization of the heterogeneous catalysta
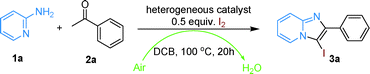
|
Entry |
Catalyst |
Isolated yield (%) |
Reaction conditions: 1a (0.6 mmol), 2a (0.5 mmol), I2 (0.25 mmol), heterogeneous catalyst (6 mg), DCB (1 mL), 100 °C, air, 20 h.
|
1 |
CuOx/OMS-2 |
93 |
2 |
CuOx–MnOx/Al–Ti |
25 |
3 |
CuOx–FeOx/OMS-2 |
59 |
4 |
CuOx–FeOx/C |
18 |
5 |
CuOx/Al–Ti |
27 |
6 |
CuOx/nano-TiO2 |
44 |
7 |
CuOx/C |
24 |
8 |
CuOx/ATP |
15 |
After confirming the superiority of CuOx/OMS-2 in this tandem reaction, the heterogeneous catalyst was characterized by TEM, XRD and XPS. Specifically, the TEM image of the catalyst showed that OMS-2 was composed of short nanorods which were structurally single crystals,21 while dispersed granular CuO was attached to the OMS-2 (Fig. 1, left). The X-ray diffraction (XRD) pattern of CuOx/OMS-2 was the same as that of the parent OMS-2 support (Fig. 1, right). No signals due to copper metal (cluster) or copper oxide were observed, which further suggested that the copper oxide was highly dispersed on the OMS-2. The X-ray photoelectron spectroscopy (XPS) profile of Cu 2p showed the banding energies of Cu 2p3/2 at 933.8 eV and Cu 2p1/2 at 954.3 eV respectively (Fig. 2, left), and the shake-up satellite peaks at around 940–944 eV, which suggests that the oxidation state of the copper species is +2.19,26 Besides, the XPS profile of Mn 2p was also demonstrated in Fig. 2 (right).19
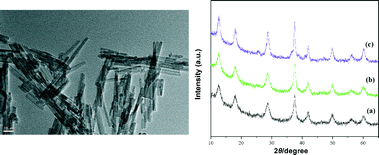 |
| Fig. 1 TEM image of CuOx/OMS-2 (left); XRD patterns (right) of (a) OMS-2, (b) CuOx/OMS-2 and (c) CuOx/OMS-2 after the first use. | |
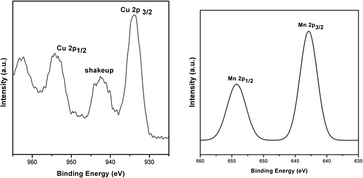 |
| Fig. 2 XPS profiles of Cu 2p (left) and Mn 2p (right). | |
To verify whether the catalysis is derived from solid CuOx/OMS-2 or leached copper species, the tandem reaction of 1a, 2a and I2 was run, and then the catalyst was removed after filtering the totally converted reaction mixture. Next, another ketone (1.0 equiv. 2-acetylthiophene) and 0.5 equiv. I2 were added into the filtrate together, and then the filtrate was treated with the rest of the 2-aminopyridine (>1.2 equiv.) under standard conditions. Consequently, only 8% of 3p was isolated, while 76% yield of 3p was obtained if fresh CuOx/OMS-2 and 0.5 equiv. I2 were added to the filtrate (Scheme 3). ICP-AES was applied for the analysis of the filtrate that was removed by CuOx/OMS-2, which confirmed that no copper species were detected in the filtrate (Cu: below 0.001%). The above results indicated that the catalysis is derived from the solid catalyst instead of the leached copper species, and that the catalyst is unambiguously heterogeneous in nature.
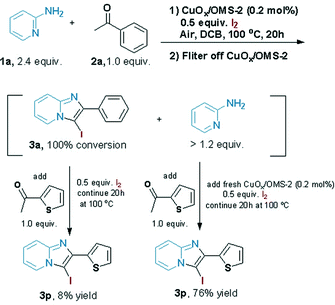 |
| Scheme 3 The test of the heterogeneous system. | |
After the completion of the tandem reaction catalyzed by CuOx/OMS-2, the catalyst was easily isolated from the reaction mixture by filtration. The XRD pattern demonstrated that the structure of the reused catalyst was preserved (Fig. 1, right) and the retrieved catalyst showed a high catalytic ability in the second run. Finally, it was found that the catalyst could be reused three times with a slight decrease in catalytic ability and that the catalytic ability could be regenerated by calcination at 350 °C for 2 h under an air atmosphere (Table 3).
Table 3 Recycling of CuOx/OMS-2a
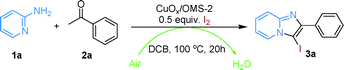
|
Reaction conditions: 2-aminopyridine (0.6 mmol), acetophenone (0.5 mmol), I2 (0.25 mmol), CuOx/OMS-2 (6 mg, 0.2 mol%), DCB (1 mL), air, 100 °C, 20 h.
Regenerated catalyst was used.
|
Cycle |
1 |
2 |
3 |
4 |
5b |
Isolated yield of 3a (%) |
93 |
89 |
81 |
69 |
90 |
The substrate scope of the reaction was examined using 0.2 mol% CuOx/OMS-2 as an efficient catalyst. Initially, 2-aminopyridine 1a and various substituted acetophenones 2 were used as substrates to expand the reaction scope under optimized reaction conditions (Table 4). Generally, substituted acetophenones with electron-donating and electron-withdrawing groups could participate in the tandem reaction efficiently, except ortho-substituted ones, like 2-Cl-acetophenone, which did not give any desired products because of steric hindrance. Specifically, electron-deficient acetophenones, including halogen and CF3, provided higher yields of the desired products than electron-rich ones, such as methyl, methoxyl, COOMe and cyano, probably because the iodination of the tandem reaction proceeded via electrophilic aromatic substitution (Table 4, 3a–3m). Furthermore, ketones containing heteroatoms reacted with 2-aminopyridine and I2 efficiently and moderate to good yields of 59–78% were obtained (Table 4, 3n–3p). However, α,β-unsaturated ketones, such as benzalacetone, did not offer any desired product at all, perhaps because the α,β-unsaturated double bond affected the iodination.
Table 4 The scope of the tandem reaction using 2-aminopyridine, I2 and various ketonesa
Next, more scope examinations were performed using different substituted 2-aminopyridines and acetophenones as raw materials (Table 5). When 3-methyl-2-aminopyridine was used as the substrate to react with electron-poor and electron-rich acetophenones, almost quantitative yields of the products were obtained (Table 5, 3q–3s). However, methylsulfonyl-substituted acetophenone only provided a moderate yield of I-substituted imidazo[1,2-a]pyridine (Table 5, 3t). Then, it was found that other substituted 2-aminopyridines, like Me–, CF3– and COOMe–, were also tolerated in this tandem reaction and gave moderate to good yields of the desired products (Table 5, 3u–3w).
Table 5 The scope of the tandem reaction using substituted 2-aminopyridines, I2 and substituted acetophenonesa
As we know, I-substituted aromatic heterocycles are versatile reaction partners for many kinds of coupling reactions since iodine is an excellent leaving group.27 Here, we report several applications of 3-iodoimidazo[1,2-a]pyridine as a versatile building block for further functionalization via coupling techniques (Scheme 4, for synthetic methods see ESI†). Firstly, the Suzuki reaction was studied using 3a and phenylboronic acid as the coupling reagents under basic Pd-catalyzed reaction conditions, and the corresponding product, 4, was isolated in 85% yield (Scheme 4a).16,28 More importantly, the direct arylation of 3-iodoimidazo[1,2-a]pyridine using an excessive amount of benzene as the arylating reagent under Pd/Cu-cocatalysis was also achieved, although the yield of the arylated product, 4, was slightly low (Scheme 4b).29 Subsequently, Ullmann-type C–N bond-formation was performed successfully using 2-aminopyridine as the aminating reagent under Ag-mediated conditions at a relatively high temperature (Scheme 4c).30 Next, an excellent yield of 2-phenyl-3-(2-phenylethynyl)H-imidazo[1,2-a]pyridine, 6, was observed when Sonogashira coupling was conducted under standard Sonogashira conditions (Scheme 4d).31 Lastly, a challenging C(sp2)–C(sp3) bond-formation was realized by using toluene as the reaction partner under Pd-catalyzed conditions (Scheme 4e). Surprisingly, the desired alkylated product, 7, was observed with the assistance of the ligand Nixantphos and excessive KN(SiMe3)2 even though the yield was not high.32
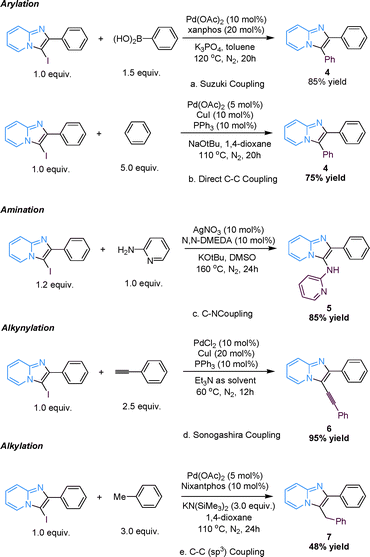 |
| Scheme 4 Late-stage functionalizations of 3-iodoimidazo[1,2-a]pyridine via various couplings. | |
To understand the mechanism of this tandem reaction, control experiments were run. If imidazo[1,2-a]pyridine, 3aa, was directly used as the raw material with I2 in DCB at 100 °C for 20 h under air, almost no 3a was isolated without adding CuOx/OMS-2. Reversely, 82% yield of 3a was obtained if 6 mg CuOx/OMS-2 was added into the reaction (Scheme 5). Additionally, CuOx/OMS-2 cannot catalyze the synthesis of imidazo[1,2-a]pyridine, 3aa, from 2-aminopyridine and acetophenone without I2 under oxidative conditions at certain temperatures.15 Therefore, the tandem reaction makes use of an I-catalyzed Ortoleva–King reaction with the assistance of Cu and a subsequent CuOx/OMS-2-catalyzed electrophilic oxidative iodination. Initially, the α-iodination of acetophenone was catalyzed by CuOx/OMS-2 using I2 as an iodinating reagent under air.33 Next, imidazo[1,2-a]pyridine was generated from α-iodoacetophenone and 2-aminopyridine via the Ortoleva–King reaction followed by cyclization, and I2 was regenerated by oxidation to finish the initial cyclization.11g,14e,15 Then, oxidative iodination of the electron-rich imidazo[1,2-a]pyridine was performed on the most acidic C–H bond via CuOx/OMS-2-catalyzed electrophilic aromatic substitution.27a,34 Eventually, the reduced copper species (I) was reoxidized by Mn(IV) to regenerate the copper species (II) and the reduced Mn was reoxidized by O2 in air via a biomimetic oxidation pathway (Scheme 1b).19
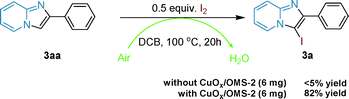 |
| Scheme 5 Control experiments. | |
Conclusions
In summary, an efficient biomimetic aerobic oxidative tandem cyclization/iodination reaction for the synthesis of 3-iodoimidazo[1,2-a]pyridines from 2-aminopyridines, acetophenones and I2, catalyzed by heterogeneous CuOx/OMS-2, was successfully developed. This tandem reaction underwent a cyclization dominated by an I-catalyzed Ortoleva–King reaction and a copper-catalyzed electrophilic oxidative iodination, which brought about 100% iodine atom economy. The tandem reactions tolerated a large range of substrates and gave moderate to great yields of products that could be applied in versatile late-stage functionalizations. Especially, CuOx/OMS-2 generated a low-energy pathway for rapid electron transfer during the oxidative process because OMS-2 played a multiple role as a support and an electron-transfer mediator (ETM), which enabled air to be the oxidant directly. Furthermore, the well-dispersed low-loading heterogeneous catalyst can be reused three times without a significant loss of catalytic ability.
Experimental
General
All reagents were purchased from commercial suppliers and used without further purification. Metal salts and catalyst supports were commercially available and were used directly. All experiments were carried out under air. Flash chromatography was carried out with Merck silica gel 60 (200–300 mesh). Analytical TLC was performed with Merck silica gel 60F254 plates, and the products were visualized by UV detection. 1H NMR and 13C NMR (400 and 100 MHz, respectively) spectra were recorded in CDCl3. Chemical shifts (δ) are reported in ppm using TMS as the internal standard, and spin-spin coupling constants (J) are given in Hz. All heterogeneous catalysts were synthesized by wet impregnation in deionized water and Cu(OH)x/OMS-2 was made by deposition/precipitation in water.
Preparation of OMS-2 (ref. 20a)
5.89 g of KMnO4 in 100 mL of deionized water was added to a solution of 8.8 g of MnSO4–H2O in 30 mL of deionized water and 3 mL of concentrated HNO3. The solution was refluxed at 100 °C for 24 h, and the product was filtered, washed, and dried at 120 °C for 8 hours. Finally, the dry OMS-2 was calcined in a muffle furnace at 350 °C for 2 hours. Then, the black powder, OMS-2, was obtained.
Preparation of CuOx/OMS-2 (ref. 20a)
The OMS-2 support (2 g) was added to a 50 mL round-bottom flask. A solution of Cu(NO3)2·3H2O (0.15 g) in deionized water (10 mL) was added to the OMS-2, and additional deionized water (10 mL) was added to wash down the sides of the flask. Then, the flask was submerged in an ultrasound bath for 3 h at room temperature and stirred for a further 20 h at room temperature. After that, the water was distilled under reduced pressure on a rotary evaporator at 80 °C for more than 2 h. Finally, the black powder was dried in an oven at 110 °C for 4 hours, followed by calcination at 350 °C for 2 hours. The Inductive Coupled Plasma Optical Emission Spectrum (ICP-OES) showed that the Cu content was 1.31 wt%.
General procedure for the CuOx/OMS-2-catalyzed tandem reaction
CuOx/OMS-2 (6 mg, 0.2 mol%), 2-aminopyridine (0.6 mmol), acetophenone (0.5 mmol), I2 (0.25 mmol) and DCB (1 mL) were added to a flask with a stir bar. The flask was stirred at 100 °C for 20 h under air. After cooling to room temperature, the mixture was diluted with ethyl acetate and filtered. The filtrate was removed under reduced pressure to obtain the crude product, which was further purified by silica gel chromatography (petroleum/ethyl acetate = 4/1 as eluent) to yield the corresponding product. The identity and purity of the products was confirmed by 1H and 13C NMR spectroscopic analysis and HRMS.
Notes and references
-
(a) M. J. Climent, A. Corma and S. Iborra, Chem. Rev., 2011, 111, 1072 CrossRef CAS PubMed;
(b) M. J. Climent, A. Corma and S. Iborra, RSC Adv., 2012, 2, 16 CAS.
- M. J. Climent, A. Corma, S. Iborra and M. J. Sabater, ACS Catal., 2014, 4, 870 CrossRef CAS.
- For selected reviews on heterogeneous catalysis, see:
(a) M. Sankar, N. Dimitratos, P. J. Miedziak, P. P. Wells, C. J. Kiely and G. J. Hutchings, Chem. Soc. Rev., 2012, 41, 8099 RSC;
(b) N. Mizuno and M. Misono, Chem. Rev., 1998, 98, 199 CrossRef CAS PubMed;
(c) E.-J. Ras and G. Rothenberg, RSC Adv., 2014, 4, 5963 RSC;
(d) J. M. Campelo, D. Luna, R. Luque, J. M. Marinas and A. A. Romero, ChemSusChem, 2009, 2, 18 CrossRef CAS PubMed;
(e)
R. A. Sheldon and H. V. Bekkum, Fine Chemicals through Heterogeneous Catalysis, Wiley-VCH, Weinheim, 2001 Search PubMed.
- For selected papers on the applications of solid-supported heterogeneous catalysts, see:
(a) X. Meng, X. Xu, T. Gao and B. Chen, Eur. J. Org. Chem., 2010, 5409 CrossRef CAS PubMed;
(b) R. N. B. Baig and R. S. Varma, Green Chem., 2013, 15, 1838 RSC;
(c) D. D. Tang, K. D. Collins and F. Glorius, J. Am. Chem. Soc., 2013, 135, 7450 CrossRef CAS PubMed;
(d) C. Liu, X. Rao, Y. Zhang, X. Li, J. Qiu and Z. Jin, Eur. J. Org. Chem., 2013, 4345 CrossRef CAS PubMed;
(e) X. Meng, C. Yu and P. Zhao, RSC Adv., 2014, 4, 8612 RSC;
(f) J. B. Bharate, S. K. Guru, S. K. Jain, S. Meena, P. P. Singh, S. Bhushan, B. Singh, S. B. Bharate and R. A. Vishwakarma, RSC Adv., 2013, 3, 20869 RSC.
-
(a) M. H. Fisher and A. Lusi, J. Med. Chem., 1972, 15, 982 CrossRef CAS;
(b) J. C. Teulade, G. Grassy, J. P. Girard and J. P. Chapat, Eur. J. Med. Chem., 1978, 13, 271 CAS.
-
(a) A. Gueiffier, M. Lhassani, A. Elhakmaoui, R. Snoeck, G. Andrei, O. Chaxignon, J. C. Teulade, A. Kerbal, E. M. Essassi, J. C. Debouzy, M. Witvrouw, Y. Blache, J. Balzarini, E. De Clercq and J. P. Chapat, J. Med. Chem., 1996, 39, 2856 CrossRef CAS PubMed;
(b) M. Lhassani, O. Chavignon, J. M. Chezal, J. C. Teulade, J. P. Chapat, R. Snoeck, G. Andrei, J. Balzarini, E. De Clercq and A. Gueiffier, Eur. J. Med. Chem., 1999, 34, 271 CrossRef CAS.
-
(a) E. Badaway and T. Kappe, Eur. J. Med. Chem., 1995, 30, 327 CrossRef;
(b) M. Hranjec, I. Piantanida, M. Kralj, L. Suman, K. Pavelıć and G. Karminski-Zamola, J. Med. Chem., 2008, 51, 4899 CrossRef CAS PubMed;
(c) M. Hranjec, M. Kralj, I. Piantanida, M. Sedıć, L. Suman, K. Pavelıć and G. Karminski-Zamola, J. Med. Chem., 2007, 50, 5696 CrossRef CAS PubMed.
- C. Hamdouchi, J. De Blas, M. del Prado, J. Gruber, B. A. Heinz and L. Vance, J. Med. Chem., 1999, 42, 50 CrossRef CAS PubMed.
-
(a) M. Baumann, I. R. Baxendale, S. V. Ley and N. Nikbin, Beilstein J. Org. Chem., 2011, 7, 442 CrossRef CAS PubMed;
(b) L. Almirante, L. Polo, A. Mugnaini, E. Provinciali, P. Rugarli, A. Biancotti, A. Gamba and W. Murmann, J. Med. Chem., 1965, 8, 305 CrossRef CAS;
(c) S. Z. Langer, Z. Arbilla, J. Benavides and B. Scatton, Adv. Biochem. Psychopharmacol., 1990, 46, 61 CAS;
(d) K. Mizushige, T. Ueda, K. Yukiiri and H. C. Suzuki, Cardiovasc. Drug Rev., 2002, 20, 163 CrossRef CAS PubMed;
(e) R. J. Boerner and H. J. Moller, Psychopharmakotherapie, 1997, 4, 145 Search PubMed.
- For selected recent papers on homogeneous synthesis of imidazopyridines using non-ketones/2-aminopyridine substrates, see:
(a) E. P. A. Talbot, M. Richardson, J. M. McKenna and F. D. Toste, Adv. Synth. Catal., 2014, 356, 687 CrossRef CAS PubMed;
(b) H. Huang, X. Ji, X. Tang, M. Zhang, X. Li and H. Jiang, Org. Lett., 2013, 15, 6254 CrossRef CAS PubMed;
(c) J. Yu, Y. Jin, H. Zhang, X. Yang and H. Fu, Chem. – Eur. J., 2013, 19, 16804 CrossRef CAS PubMed;
(d) K. B. Puttaraju and K. Shivashankar, RSC Adv., 2013, 3, 20883 RSC;
(e) N. Chernyak and V. Gevorgyan, Angew. Chem., 2010, 122, 2803 (
Angew. Chem., Int. Ed.
, 2010
, 49
, 2743
) CrossRef PubMed;
(f) I. R. Siddiqui, P. Rai, Rahila, A. Srivastava and S. Shamim, Tetrahedron Lett., 2014, 55, 1159 CrossRef CAS PubMed.
-
(a) E. S. Hand and W. W. Paudler, J. Org. Chem., 1978, 43, 2900 CrossRef CAS;
(b) N. Denora, V. Laquintana, M. G. Pisu, R. Dore, L. Murru, A. Latrofa, G. Trapani and E. Sanna, J. Med. Chem., 2008, 51, 6876 CrossRef CAS PubMed;
(c) D. Zhu, J. Chen, D. Wu, M. Liu, J. Ding and H. Wu, J. Chem. Res., 2009, 2, 84 CrossRef;
(d) D.-J. Zhu, J.-X. Chen, M.-C. Liu, J.-C. Ding and H.-Y. Wu, J. Braz. Chem. Soc., 2009, 20, 482 CrossRef CAS PubMed;
(e) S. E. Kazzouli, S. Berteina-Raboin, A. Mouaddib and G. Guillaumet, Tetrahedron Lett., 2003, 44, 6265 CrossRef;
(f) M. Ueno, T. Nabana and H. Togo, J. Org. Chem., 2003, 68, 6424 CrossRef CAS PubMed;
(g) A. J. Stasyuk, M. Banasiewicz, M. K. Cyrański and D. T. Gryko, J. Org. Chem., 2012, 77, 5552 CrossRef CAS PubMed;
(h) T. J. Donohoe, M. A. Kabeshov, A. H. Rathi and I. E. D. Smith, Org. Biomol. Chem., 2012, 10, 1093 RSC;
(i) Z.-G. Le, Z.-B. Xie and J.-P. Xu, Molecules, 2012, 17, 13368 CrossRef CAS PubMed;
(j) J. S. Yadav, B. V. Subba Reddy, Y. Gopal Rao, M. Srinivas and A. V. Narsaiah, Tetrahedron Lett., 2007, 43, 7717 CrossRef PubMed;
(k) Y.-Y. Xue, Z.-C. Chen and Q.-G. Zheng, Synthesis, 2002, 11, 1505 Search PubMed;
(l) S. Kumar and D. P. Sahu, ARKIVOC, 2008, xv, 88 CrossRef;
(m) A. R. Katritzky, D. O. Tymoshenko, D. Monteux, V. Vvedensky, G. Nikonov, C. B. Cooper and M. Deshpande, J. Org. Chem., 2000, 65, 8059 CrossRef CAS PubMed.
- C. He, J. Hao, H. Xu, Y. Mo, H. Liu, J. Han and A. Lei, Chem. Commun., 2012, 48, 11073 RSC.
- S. Santra, A. K. Bagdi, A. Majee and A. Hajra, Adv. Synth. Catal., 2013, 355, 1065 CrossRef CAS PubMed.
-
(a) A. K. Bagdi, M. Rahman, S. Santra, A. Majee and A. Hajra, Adv. Synth. Catal., 2013, 355, 1741 CrossRef CAS PubMed;
(b) D. C. Mohan, R. R. Donthiri, S. N. Rao and S. Adimurthy, Adv. Synth. Catal., 2013, 355, 2217 CrossRef PubMed;
(c) Z.-J. Cai, S.-Y. Wang and S.-J. Ji, Adv. Synth. Catal., 2013, 355, 2686 CrossRef CAS PubMed;
(d) K. Pericherla, P. Kaswan, P. Khedar, B. Khungar, K. Parang and A. Kumar, RSC Adv., 2013, 3, 18923 RSC;
(e) Y. Zhang, Z. Chen, W. Wu, Y. Zhang and W. Su, J. Org. Chem., 2013, 78, 12494 CrossRef CAS PubMed.
- X. Meng, Y. Wang, C. Yu and P. Zhao, RSC Adv., 2014, 4, 27301 RSC.
- Y. Gao, M. Yin, W. Wu, H. Huang and H. Jiang, Adv. Synth. Catal., 2013, 355, 2263 CrossRef CAS PubMed.
- For a review on biomimetic aerobic oxidation, see: J. Piera and J. E. Bäckvall, Angew. Chem., 2008, 120, 3558 (
Angew. Chem., Int. Ed.
, 2008
, 47
, 3506
) CrossRef PubMed.
- For selected papers on biomimetic oxidation catalyzed by transition metals and ETMs, see:
(a) B. P. Babu, X. Meng and J. E. Bäckvall, Chem. – Eur. J., 2013, 19, 4140 CrossRef CAS PubMed;
(b) Y. Endo and J. E. Bäckvall, Chem. – Eur. J., 2011, 17, 12596 CrossRef CAS PubMed;
(c) B. P. Babu, Y. Endo and J. E. Bäckvall, Chem. – Eur. J., 2012, 18, 11524 CrossRef CAS PubMed;
(d) M. Johansson, B. W. Purse, O. Terasaki and J. E. Bäckvall, Chem. – Eur. J., 2008, 350, 1807 CAS;
(e) N. Gigant and J. E. Bäckvall, Org. Lett., 2014, 16, 1664 CrossRef CAS PubMed;
(f) N. Gigant and J. E. Bäckvall, Chem. – Eur. J., 2013, 19, 10799 CrossRef CAS PubMed;
(g) X. Meng, C. Li, B. Han, T. Wang and B. Chen, Tetrahedron, 2010, 66, 4029 CrossRef CAS PubMed.
- T. Oishi, K. Yamaguchi and N. Mizuno, ACS Catal., 2011, 1, 1351 CrossRef CAS.
-
(a) R. N. DeGuzman, Y.-F. Shen, E. J. Neth, S. L. Suib, C.-L. O'Young, S. Levine and J. M. Newsam, Chem. Mater., 1994, 6, 815 CrossRef CAS;
(b) Y. F. Shen, R. P. Zerger, R. N. DeGuzman, S. L. Suib, L. McCurdy, D. I. Potter and C. L. O'Young, Science, 1993, 260, 511 CAS;
(c) S. L. Suib, J. Mater. Chem., 2008, 18, 1623 RSC;
(d) S. L. Suib, Acc. Chem. Res., 2008, 41, 479 CrossRef CAS PubMed.
- For selected recent papers on OMS-2 catalysis, see:
(a) J. R. Kona, C. K. Kingòndu, A. R. Howell and S. L. Suib, ChemCatChem, 2014, 6, 749 CrossRef CAS PubMed;
(b) K. Yamaguchi, H. Kobayashi, T. Oishi and N. Mizuno, Angew. Chem., 2012, 124, 559 (
Angew. Chem., Int. Ed.
, 2012
, 51
, 544
) CrossRef PubMed;
(c) K. Yamaguchi, Y. Wang and N. Mizuno, ChemCatChem, 2013, 5, 2835 CrossRef CAS PubMed;
(d) K. Yamaguchi, H. Kobayashi, Y. Wang, T. Oishi, Y. Ogasawara and N. Mizuno, Catal. Sci. Technol., 2013, 3, 318 RSC;
(e) Y. Wang, H. Kobayashi, K. Yamaguchi and N. Mizuno, Chem. Commun., 2012, 48, 2642 RSC;
(f) L. Jin, J. Reutenauer, N. Opembe, M. Lai, D. J. Martenak, S. Han and S. L. Suib, ChemCatChem, 2009, 1, 441 CrossRef CAS PubMed;
(g) S. Dharmarathna, C. K. Kingòndu, W. Pedrick, L. Pahalagedara and S. L. Suib, Chem. Mater., 2012, 24, 705 CrossRef CAS;
(h) N. N. Opembe, Y. C. Son, T. Sriskandakumar and S. L. Suib, ChemSusChem, 2008, 1, 182 CrossRef CAS PubMed;
(i) R. Kumar, S. Sithambaram and S. L. Suib, J. Catal., 2009, 262, 304 CrossRef CAS PubMed;
(j) S. Sithambaram, L. Xu, C. H. Chen, Y. Ding, R. Kumar, C. Calvert and S. L. Suib, Catal. Today, 2009, 140, 162 CrossRef CAS PubMed;
(k) X. Jin, K. Yamaguchi and N. Mizuno, RSC Adv., 2014, 4, 34712 RSC.
- For selected papers on OMS-2 used as a support for heterogeneous catalysis, see:
(a) X. Jin, K. Yamaguchi and N. Mizuno, Angew. Chem., 2014, 126, 465 (
Angew. Chem., Int. Ed.
, 2014
, 53
, 455
) CrossRef PubMed;
(b) K. Yamaguchi, Y. Wang, T. Oishi, Y. Kuroda and N. Mizuno, Angew. Chem., 2013, 125, 5737 (
Angew. Chem., Int. Ed.
, 2013
, 52
, 5627
) CrossRef PubMed.
- The data for the redox potentials (in water at pH = 0) were obtained from the following reference: M. Pourbaix, Atlas of Electrochemical Equilibria in Aqueous Solutions, NACE, Houston, 1966 Search PubMed.
-
(a) J. Liu, C. Yu, P. Zhao and G. Chen, Appl. Surf. Sci., 2012, 258, 9096 CrossRef CAS PubMed;
(b) C. Yu, P. Zhao, G. Chen and B. Hu, Appl. Surf. Sci., 2011, 257, 7727 CrossRef CAS PubMed.
- Z. Fei, Y. Zhu, M. Liu, F. Jia and A. Wu, Tetrahedron Lett., 2013, 54, 1222 CrossRef CAS PubMed.
- J.-L. Cao, G.-S. Shao, Y. Wang, Y. Liu and Z.-Y. Yuan, Catal. Commun., 2008, 9, 2555 CrossRef CAS PubMed.
-
(a) S. Stavber, M. Jereb and M. Zupan, Synthesis, 2008, 10, 1487 CrossRef;
(b) F. Monnier and M. Taillefer, Angew. Chem., 2009, 121, 7088 (
Angew. Chem., Int. Ed.
, 2009
, 48
, 6954
) CrossRef PubMed;
(c) S. V. Ley and A. W. Thomas, Angew. Chem., 2003, 115, 5558 (
Angew. Chem., Int. Ed.
, 2003
, 42
, 5400
) CrossRef PubMed.
-
(a) A. Suzuki, Angew. Chem., 2011, 123, 6854 (
Angew. Chem., Int. Ed.
, 2011
, 50
, 6723
) CrossRef PubMed;
(b) E. Negishi, Angew. Chem., 2011, 123, 6870 (
Angew. Chem., Int. Ed.
, 2011
, 50
, 6738
) CrossRef PubMed.
- D. Alberico, M. E. Scott and M. Lautens, Chem. Rev., 2007, 107, 174 CrossRef CAS PubMed.
- C. Sambiagio, S. P. Marsden, A. J. Blacker and P. C. McGowan, Chem. Soc. Rev., 2014, 43, 3525 RSC.
-
(a) K. Sonogashira, Y. Tohda and N. Hagihara, Tetrahedron Lett., 1975, 4467 CrossRef CAS;
(b) R. Chinchilla and C. Nájera, Chem. Rev., 2007, 107, 874 CrossRef CAS PubMed.
- A. D. Creamer, S. D. Dreher and P. J. Walsh, J. Am. Chem. Soc., 2012, 134, 13765 CrossRef PubMed.
- G. Yin, M. Gao, N. She, S. Hu, A. Wu and Y. Pan, Synthesis, 2007, 20, 3113 Search PubMed.
-
(a) H.-Q. Do and O. Daugulis, J. Am. Chem. Soc., 2011, 133, 13577 CrossRef CAS PubMed;
(b) H.-Q. Do and O. Daugulis, Org. Lett., 2009, 11, 421 CrossRef CAS PubMed;
(c) I. Popov, H.-Q. Do and O. Daugulis, J. Org. Chem., 2009, 74, 8309 CrossRef CAS PubMed.
Footnote |
† Electronic supplementary information (ESI) available: Experimental details, spectral data of the products and copies of the spectra. See DOI: 10.1039/c4cy00919c |
|
This journal is © The Royal Society of Chemistry 2015 |
Click here to see how this site uses Cookies. View our privacy policy here.