DOI:
10.1039/C6RA26085C
(Paper)
RSC Adv., 2017,
7, 678-683
Quantum size effect and catalytic activity of nanosized single-crystalline spherical β-Ga2O3 particles by thermal annealing of liquid metal nanoparticles†
Received
31st October 2016
, Accepted 28th November 2016
First published on 28th November 2016
Abstract
We report on the synthesis of nanosized single-crystalline spherical β-Ga2O3 particles (GONP) by thermal annealing of gallium nanoparticles (GaNP), which previously has been prepared under ultrasonication. GONP displayed a spherical morphology and the particle size reflected that of GaNP. The nanoparticles have been confirmed as crystalline β-Ga2O3 by powder X-ray diffraction. The selected area electron diffraction patterns obtained from a whole particle of one GONP represented production of single-crystalline nanoparticle. Furthermore, the dispersibility and stability of GONP in solvent dramatically improved through silica coating (GO@SiO2 NP). Photoluminescence spectroscopy indicated that the GO@SiO2 NP exhibited a light blue emission at 445 nm under excitation at 240 nm. Diffuse reflectance spectroscopy demonstrated that the band gap of GO@SiO2 NP (Eg = 4.89 eV) was hypsochromically shifted compared to that of bulk Ga2O3 (Eg(bulk) = 4.56 eV) due to the quantum size effect of β-Ga2O3. Additionally, silica particle-supported GONP (GONP/MCM-41) showed catalytic activity for the benzylation of benzene with benzyl chloride. Thus, the GONP presented here will have potential applications in LED phosphors, optoelectronic devices, and supported catalysts.
Introduction
Monoclinic gallium oxide (β-Ga2O3) is a well-known wide-band-gap semiconductor (Eg > 4.4 eV) that exhibits luminescence and conduction properties.1–4 Due to these unique performances, β-Ga2O3 can be used for a variety of applications including electronic and optoelectronic devices, such as high sensitivity gas sensors, and transparent conductors.5–10 Up to now, micrometer/nanometer scaled single-crystalline β-Ga2O3 with varied morphology, for instance, rods, wires, tubes, plates have been synthesized and characterized.11–19 However, examples of the preparation of single-crystalline spherical β-Ga2O3 particles has still remained very few. Recently, Tang et al. have reported a simple method to prepare submicrometer-sized spherical β-Ga2O3 particles with uniform diameters.20 The β-Ga2O3 submicron particle was synthesized by heating molten gallium, and the resulting particles exhibited a broad emission spectrum with a maximum at 458 nm under 260 nm excitation, since various quantum size effects in electronic, optical, and/or magnetic properties, such as an increase in the band gap of a semiconducting material with a reduction of the size, are generally observed for other materials.21–27 Downsizing of β-Ga2O3 submicron particle will also provide distinctive quantum size effect on these properties. Nevertheless, to the best of our knowledge, preparation of single-crystalline spherical β-Ga2O3 nanoparticles and the quantum size effect have been never reported yet.
These facts prompted us to explore the quantum size effect of β-Ga2O3 particles. We previously reported preparation of liquid-metal nanocolloid composed of liquid gallium, solvents, and surfactants with ultrasonic irradiation.28 The direct synthesis method enabled to control the size of gallium nanoparticle (GaNP) by tuning the surfactants, temperature, and ultrasonic irradiation intensity.
In this report, we demonstrate for the first time preparation of single-crystalline spherical β-Ga2O3 nanoparticle (GONP) derived from GaNP as precursor (Fig. 1). To suppress the aggregation of prepared GONP, we selected silica coated GaNP (Ga@SiO2 NP) as precursors of GONP. The obtained GONP exhibited luminescence property and hypsochromic shift of band gap due to quantum size effect of β-Ga2O3. Furthermore, the GONP were supported on MCM-41, resulting in catalytic activity for Friedel–Crafts type benzylation.
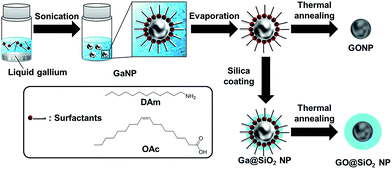 |
| Fig. 1 Schematic illustration of preparation of spherical β-Ga2O3 nanoparticle (GONP) and silica-coated β-Ga2O3 nanoparticle (GO@SiO2 NP) from gallium nanoparticle (GaNP) as precursors. Surfactants are dodecylamine (DAm) or oleic acid (OAc). | |
Experimental section
Materials and instrumentation
Gallium (high purity, 99.9999%, Mitsuwa Chemicals Co., Ltd.), dodecylamine (Tokyo Chemical Industry Co., Ltd.), oleic acid (Wako chemical industries, Ltd.), tetramethyl orthosilicate (Tokyo Chemical Industry Co., Ltd.), chloroform (Wako chemical industries, Ltd.), 2-propanol (Wako chemical industries, Ltd.) were purchased from each company, and used without further purification. Sonication was carried out with ELMA Elmasonic S30H and SND US-2KS (80 W for GaNPs@DAm and 120 W for GaNPs@OAc). Powder X-ray diffraction (XRD) patterns were obtained by Bruker AXS D8 ADVANCE. Transmission electron microscopy (TEM) was conducted on a JEOL JEM-2100F having EDX function and a JEOL JEM-2010 for selected-area electron diffraction (SAED). Emission spectra were obtained with SHIMADZU RF5300PC spectrofluorometer. UV-Vis spectra were recorded on a JASCO V-570 spectrophotometer. 1H (500 MHz) NMR spectra were measured on a Bruker Biospin AVANCE DRX500 instrument, using 0.05% tetramethylsilane (TMS) as an internal standard. TEM observation was conducted at the OPEN FACILITY, Hokkaido University Sousei Hall.
Preparation of GaNP suspension28
Metallic gallium (100 mg, 1.4 mmol) was added to a chloroform solution (2.4 mL) of dodecylamine (276 μL), and the solution was heated at 80 °C for 15 min. After visual confirmation of gallium melting, the solution was sonicated by a bath type sonicator at 0 °C for 10 min, which resulted in dodecylamine stabilized GaNP (GaNP@DAm) suspension.
Preparation of GONP
GaNP@DAm suspension was separated by centrifugation, and the GaNP@DAm powder was obtained by drying. GONP was prepared by thermal annealing under air using electric furnace and oven at 500–900 °C under ambient atmosphere for 1 hour.
Preparation of SiO2 coated GaNP (Ga@SiO2 NP)
Metallic gallium (100 mg, 1.4 mmol) was added to a chloroform solution (2.4 mL) of oleic acid (381 μL), and the solution was heated at 80 °C for 15 min. After confirmation of melting gallium, the solution was sonicated by bath type sonicator at 0 °C for 10 min, which resulted in oleic acid stabilized GaNP (GaNP@OAc) suspension. GaNP@OAc suspension was separated by centrifugation, and the GaNP@OAc powder was obtained by drying. Then, the GaNP@OAc powder was added to 90 mL 2-propanol, 7.2 mL water, and 900 μL ammonia solution. Under continuous stirring, 0.9 mg of tetramethyl orthosilicate (TMOS) was added to the reaction mixture. The reaction was allowed to proceed for 1 hour at room temperature under continuous stirring, which resulted in silica coated GaNP@OAc (Ga@SiO2 NP) suspension.
Preparation of silica coated GONP (GO@SiO2 NP)
Ga@SiO2 NP suspension was separated by centrifugation and Ga@SiO2 NP powder were obtained by drying. Silica coated GONP (GO@SiO2 NP) was prepared by thermal annealing using electric furnace and oven at 700 °C for 1 hour.
Preparation of MCM-41 supported GONP (GONP/MCM-41)
GONP supported on MCM-41 (GONP/MCM-41) was synthesized by evaporation to dryness. MCM-41 (20 mg) was dispersed in chloroform (6 mL). Then, GaNP@OAc powder (2 mg) was added to MCM-41 dispersion. After ultrasonication, the mixture was evaporated to dryness, which resulted in GaNP@OAc supported on MCM-41. Supported GONP was prepared from GaNP@OAc supported on MCM-41 by thermal annealing using electric furnace and oven at 700 °C for 1 hour.
Evaluation for catalytic activity of supported GONP
The liquid phase benzylation of benzene with benzyl chloride (BzCl) was selected as a probe reaction for studying the activity of catalysts. The reaction was carried out in a 25 mL round bottomed flask under continuous stirring equipped with condenser, a nitrogen inlet for maintaining an inert atmosphere. The temperature was maintained in oil bath and the reaction carried out at selected reaction condition with reaction temperature of 353 K, benzene to BzCl molar ratio of 10, and the catalyst amount of 1 wt% of the total reaction mixture. After 3 hours, the sample was withdrawn and analyzed with 1H NMR measurement. The conversion was based on the consumed in the reaction mixture.
Results and discussion
The GONP powder was obtained from GaNP@DAm after annealing under air for 1 hour at various temperatures. In the case of annealing temperature above 700 °C, the obtained powder was colourless, while that annealed below 600 °C exhibited gray color. Their crystal phase was identified by XRD, as shown Fig. 2. Whereas the XRD patterns of GONP powders treated above 700 °C confirms that most of the strong peaks corresponded with commercial β-Ga2O3, powders treated below 600 °C reveal low crystalline nature. This fact indicated the importance of annealing temperature on the crystallinity of GONP.
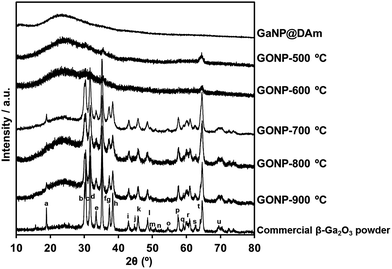 |
| Fig. 2 XRD patterns of the powders annealed with different temperatures of GaNP@DAm before annealing, GONP-500 °C, GONP-600 °C, GONP-700 °C, GONP-800 °C, GONP-900 °C, and commercial β-Ga2O3 powder. a: (−201), b: (400), c: (−401), d: (002), (−202), e: (−111), f: (111), g: (401), h: (−311), (−402), (202), i: (−112), j: (−601), k: (600), (−312), (112), l: (510), m: (402), n: (−403), o: (511), (203), p: (−313), q: (113), r: (−801), s: (710), t: (403), (512), (−222), u: (−421), (022), (−222). | |
The morphology of products obtained after thermal annealing with various temperatures was investigated by transmission electron microscope (TEM) as shown Fig. 3. It clearly shows nanosized spherical particles treated below 700 °C (Fig. 3b–d), derived from the original GaNP@DAm particle (Fig. 3a). Indeed, the measured particle distribution of GONP annealed at 700 °C (15.9 ± 4.2 nm) was similar with that of GaNP@DAm (13.3 ± 4.9 nm, Fig. S1†), while the GONP partially aggregated to form larger particle (Fig. 3d). With the increase of thermal annealing temperature, the particle was likely to aggregate and formed bulky crystal with irregular morphologies, probably due to due to higher oxidation reaction rate (Fig. 3e and f).
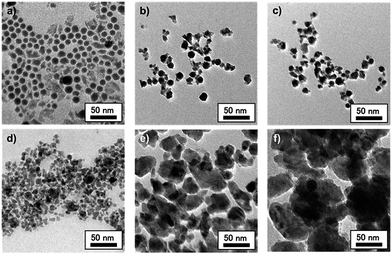 |
| Fig. 3 TEM images of (a) GaNP@DAm before annealing, and GONP annealed at (b) 500 °C, (c) 600 °C, (d) 700 °C, (e) 800 °C, and (f) 900 °C. | |
High magnification TEM images of a single particle annealed at various temperatures are shown in Fig. 4a–e. The crystalline nature of these particles was verified by the high-resolution TEM (HRTEM), as shown in Fig. 4f–j. The marked interplanar d-spacing of all samples corresponded with facet of β-Ga2O3 such as (403), (400) or (111). However for particles treated below 600 °C, the mixed population of crystalline part and amorphous part in a single particle was observed (Fig. 4f and g). Thus, crystallization of GONP was completely proceeded above 700 °C.29
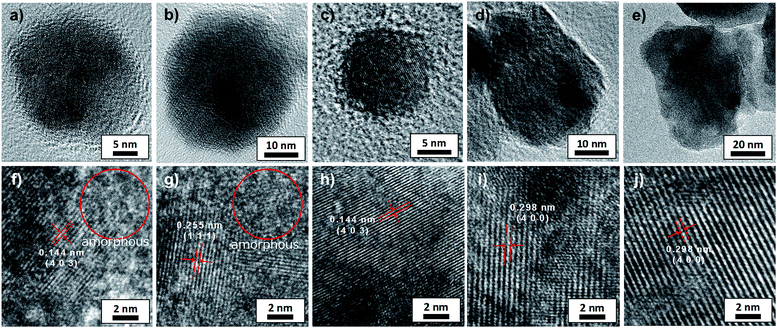 |
| Fig. 4 TEM images of single particle of GONP annealed at different temperatures: (a) 500 °C, (b) 600 °C, (c) 700 °C, (d) 800 °C, and (e) 900 °C. HRTEM images of single particle prepared at different temperatures: (f) 500 °C, (g) 600 °C, (h) 700 °C, (i) 800 °C, and (j) 900 °C. | |
In order to confirm its single-crystalline character of the particles treated above 700 °C, we obtained selected-area electron diffraction (SAED) pattern from a single particle (Fig. 5). From the SAED patterns of single particle annealed at 700 °C, all the diffraction spots can be indexed to single-crystalline β-Ga2O3 (Fig. 5a). On the other hand, the SAED patterns of single particle annealed at 900 °C revealed poly crystalline β-Ga2O3 as can be seen in the unindexed spots marked by yellow circle in Fig. 5b.
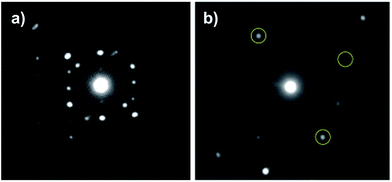 |
| Fig. 5 SAED patterns of single particle of GONP annealed at (a) 700 °C, and (b) 900 °C. | |
The result of preparation of GONP was summarized in Table 1. These observations offered two facts for preparation of GONP. At first, when thermal annealing temperature was 500 °C or 600 °C, crystallization of β-Ga2O3 was not completely proceeded with the result that gallium and β-Ga2O3 mixed nanoparticles was formed. In the case of thermal annealing temperature above 700 °C, gallium part of GaNP@DAm as precursors perfectly transformed into β-Ga2O3, resulted in formation of single-crystalline GONP at 700 °C. However for the second fact, aggregation and fusion of single-crystalline GONP was occurred at 800 °C or 900 °C, which brought about the formation of polycrystalline GONP.
Table 1 The characteristics of GaNP@DAm and GONP prepared under various condition
Sample |
Color |
Shape |
Composition |
Crystallinity |
GaNP@DAm
|
Black |
Sphere |
Amorphous |
— |
GONP-500 °C |
Gray |
Sphere |
Amorphous + crystal |
— |
GONP-600 °C |
Gray |
Sphere |
Amorphous + crystal |
— |
GONP-700 °C |
White |
Sphere |
Crystal |
Single crystal |
GONP-800 °C |
White |
Non-sphere |
Crystal |
Poly crystal |
GONP-900 °C |
White |
Non-sphere |
Crystal |
Poly crystal |
Then we focused characteristic and property of single-crystalline GONP annealed at 700 °C. We investigated the emission property of the GONP. The absorption, excitation, and emission spectra of the GONP dispersed in methanol were measured at room temperature, as shown in Fig. 6. The emission spectrum shows a broad light blue emission band centered at 451 nm under a 248 nm excitation. Note that GONP prepared at 900 °C exhibited the emission maximum at 470 nm, which was identical to that of commercial β-Ga2O3 powder, indicative of the importance of single crystalline nature for the emission property. The band gap (Eg) of GONP was estimated by using a Tauc plot of UV-Vis diffuse reflectance spectroscopy (Fig. 6b), which was found to be 4.84 eV. The commercial β-Ga2O3 powder showed its band gap at 4.56 eV, thus a quantum size effect of β-Ga2O3 caused the 0.28 eV of hypsochromic shift on the band gap, corresponding to the anticipated value from Brus equation (Fig. S2†).30,31
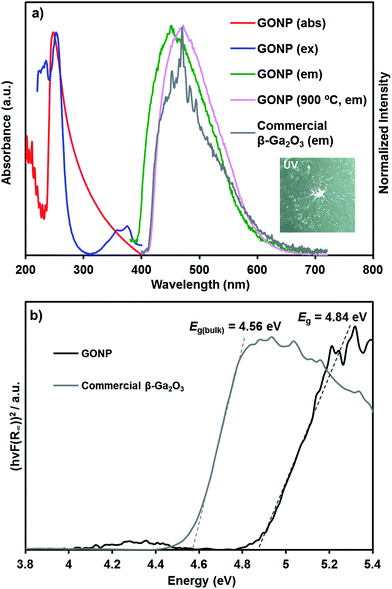 |
| Fig. 6 (a) Absorption, excitation (λem = 451 nm) and emission spectra (λex = 248 nm) of single-crystalline GONP and commercial β-Ga2O3 powder. Emission spectrum of GONP prepared at 900 °C was also shown. Inset shows the photograph of single-crystalline GONP under UV irradiation at 254 nm. (b) Band gap data of single-crystalline GONP and commercial β-Ga2O3 powder obtained from UV reflectance. | |
The obtained single crystalline GONP slightly aggregated due to disappearance of stabilizer through thermal annealing, as shown in Fig. 3d. To suppress aggregation of GONP by coating GaNP with heat-resistant materials, we attempted to prepare silica coated GONP (GO@SiO2 NP) utilizing silica coated GaNP (Ga@SiO2 NP) as precursors to suppress aggregation of nanoparticles. Moreover, we selected smaller GaNP (GaNP@OAc; average diameter: ca. 8 nm) compared to GaNP@DAm (average diameter: ca. 15 nm) as the precursor. Ga@SiO2 NP was obtained through a sol–gel reaction of tetramethyl orthosilicate (TMOS) catalyzed by aqueous ammonia as a catalyst in alcoholic solvent at room temperature. An excess amount of TMOS was employed due to the in stability of GaNP in an alcoholic solution. After the sol–gel reaction for 1 hour, GO@SiO2 NP was prepared by thermal annealing at 700 °C for 1 hour. Thermal annealing caused colour change of powder from black to white. Whereas the XRD patterns of Ga@SiO2 NP powder shows amorphous, powders treated at 700 °C confirms weak peaks at 35.3° and 64.3°, respectively attributed to (111) and (403) of β-Ga2O3 (Fig. 7).
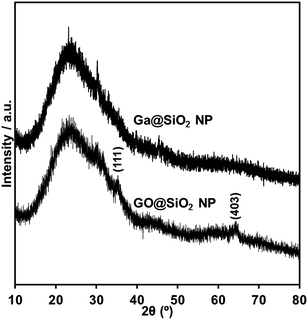 |
| Fig. 7 XRD patterns of silica coated GaNP@OAc (Ga@SiO2 NP) and silica coated GONP (GO@SiO2 NP). | |
TEM observation of resulted particles revealed the formation of GO@SiO2 NP, which showed similar appearance with Ga@SiO2 NP (Fig. 8a and b). The distribution of particle diameter in GO@SiO2 NP (9.1 ± 1.6 nm) was close to that of Ga@SiO2 NP (9.6 ± 1.8 nm, Fig. S3†). EDX-analysis of GO@SiO2 NP proved the existence of Ga and Si atoms as well as those in Ga@SiO2 NP. Then we investigated the emission property and bang gap of GO@SiO2 NP. Analogous to GONP, emission spectrum of GO@SiO2 NP showed a broad emission spectrum centered at 446 nm under a 240 nm excitation, which was caused hypsochromic shift compared with GONP (Fig. S4†). Furthermore, the band gap of GO@SiO2 NP was determined as 4.89 eV from Tauc plot, thus larger than that of GONP (Fig. 6b). These results confirmed existence of quantum size effect of β-Ga2O3.
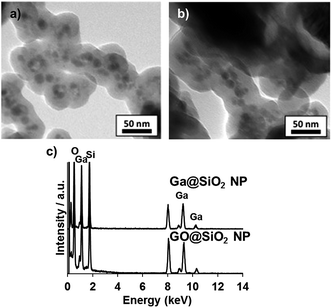 |
| Fig. 8 TEM images of (a) Ga@SiO2 NP, and (b) GO@SiO2 NP, and (c) TEM-EDX analysis of Ga@SiO2 NP and GO@SiO2 NP. | |
To investigate the catalytic activity of GONP, benzylation of benzene with benzyl chloride (BzCl) has been carried out.32–34GONP/MCM-41 was used for benzylation at the reaction temperature of 80 °C, the reaction time of 3 hours and the benzene to BzCl ratio 10 and the results are shown Fig. 9. The major product in the reaction is diphenylmethane (DPM). The trace of the formation of by-product (dibenzylbenzene) was found. BzCl conversion and DPM selectivity were calculated to be 71% and 51%, respectively. The time of frequency (TOF) of this catalysis was found to be 25 h−1. On the other hand, commercial β-Ga2O3 powder did not show formation of DPM at all. This result revealed that our catalyst was active for the benzylation of benzene with BzCl, probably due to the increased surface area of β-Ga2O3.
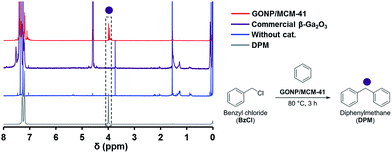 |
| Fig. 9
1H NMR spectra of sample after benzylation under various conditions. | |
Conclusions
We have demonstrated a novel approach to prepare nanosized β-Ga2O3 particles utilizing GaNP as precursors. A strong dependence between the structure of GONP and the thermal annealing temperature was observed. Furthermore, it was found that silica coating allowed to maintain the spherical shape of nanoparticles without formation of aggregation and change of morphology (GO@SiO2 NP). The single-crystalline GONP with spherical morphology exhibited a light blue emission and band gap shift derived from quantum size effect compared to bulk β-Ga2O3. Moreover, MCM-41 supported GONP (GONP/MCM-41) revealed catalytic activity for benzylation of benzene with BzCl. We strongly believe that GONP will be suitable for applications in LED phosphors, optoelectronic devices, and supported catalysts.
Acknowledgements
The authors acknowledge financial support from a JSPS Grant-in-Aid for Scientific Research (B) (26288054) and a JSPS Grant-in-Aid for Young Scientists (B) (15K17861). The authors appreciate Prof. M. Kato and Prof. Y. Wang for XRD measurements and TEM (environmental cell) for SAED, respectively. The TEM observation was carried out at the OPEN FACILITY, Hokkaido University Sousei Hall.
Notes and references
- H. H. Tippins, Phys. Rev. [Sect.] A, 1965, 140, A316–A319 CrossRef.
- M. Passlack, E. F. Schubert, W. S. Hobson, M. Hong, N. Moriya, S. N. G. Chu, K. Konstadinis, J. P. Mannaerts, M. L. Schnoes and G. Zydzik, J. Appl. Phys., 1995, 77, 686–693 CrossRef CAS.
- K. Yamaguchi, Solid State Commun., 2004, 131, 739–744 CrossRef CAS.
- H. He, R. Orlando, M. A. Blanco, R. Pandey, E. Amzallag, I. Baraille and M. Rerat, Phys. Rev. B: Condens. Matter Mater. Phys., 2006, 74, 195123 CrossRef.
- M. Passlack, M. Hong and J. P. Mannaerts, Appl. Phys. Lett., 1996, 68, 1099 CrossRef CAS.
- Z. Li, C. Groot, J. Jagadeesh and H. Moodera, Appl. Phys. Lett., 2000, 77, 3630 CrossRef CAS.
- L. Binet and D. Gourier, J. Phys. Chem. Solids, 1998, 59, 1241–1249 CrossRef CAS.
- T. Minami, MRS Bull., 2000, 8, 38–44 CrossRef.
- M. Ogita, N. Saika, Y. Nakanishi and Y. Hatanaka, Appl. Surf. Sci., 1999, 142, 188–191 CrossRef CAS.
- P. Feng, J. Y. Zhang, Q. H. Li and T. H. Wang, Appl. Phys. Lett., 2006, 88, 153107 CrossRef.
- X. C. Wu, W. H. Song, W. D. Huang, M. H. Pu, B. Zhao, Y. P. Sun and J. J. Du, Chem. Phys. Lett., 2000, 328, 5–9 CrossRef CAS.
- Y. C. Choi, W. S. Kim, Y. S. Park, S. M. Lee, D. J. Bae, Y. H. Lee, G. S. Park, W. B. Choi, N. S. Lee and J. M. Kim, Adv. Mater., 2000, 12, 746–750 CrossRef CAS.
- S. Sharma and M. K. Sunkara, J. Am. Chem. Soc., 2002, 124, 12288–12293 CrossRef CAS PubMed.
- Y. P. Song, H. Z. Zhang, C. Lin, Y. W. Zhu, G. H. Li, F. H. Yang and D. P. Yu, Phys. Rev. B: Condens. Matter Mater. Phys., 2004, 69, 075304 CrossRef.
- P. C. Chang, Z. Fan, W. Y. Tseng, A. Rajagopal and J. G. Lu, Appl. Phys. Lett., 2005, 87, 222102 CrossRef.
- J. Hu, Q. Li, J. Zhan, Y. Jiao, Z. Liu, S. P. Ringer, Y. Bando and D. Golberg, ACS Nano, 2008, 2, 107–112 CrossRef CAS PubMed.
- Y. Huang, Z. Wang, Q. Wang, C. Gu, C. Tang, Y. Bando and D. Golberg, J. Phys. Chem. C, 2009, 113, 1980–1983 CAS.
- S. Yan, L. Wan, Z. Li, Y. Zhou and Z. Zou, Chem. Commun., 2010, 46, 6388–6390 RSC.
- J. Lin, Y. Huang, Y. Bando, C. Tang, C. Li and D. Golberg, ACS Nano, 2010, 4, 2452–2458 CrossRef CAS PubMed.
- T. Zhang, J. Lin, X. Zhang, Y. Huang, X. Xu, Y. Xue, J. Zou and C. Tang, J. Lumin., 2013, 140, 30–37 CrossRef CAS.
- V. I. Klimov, A. A. Mikhailovsky, S. Xu, A. Malko, J. A. Hollingsworth, C. A. Leatherdale, H. J. Eisler and M. G. Bawendi, Science, 2000, 290, 314–317 CrossRef CAS PubMed.
- A. V. Dijken, E. A. Meulenkamp, D. Vanmaekelbergh and A. Meijerink, J. Lumin., 2000, 90, 123–128 CrossRef.
- P. Claus, A. Brückner, C. Mohr and H. Hofmeister, J. Am. Chem. Soc., 2000, 122, 11430–11439 CrossRef CAS.
- Y. Volokitin, J. Sinzig, L. J. Jongh, G. Schmid, M. N. Vargaftik and I. I. Moiseev, Nature, 1996, 384, 621–623 CrossRef CAS.
- R. Viswanatha, S. Sapra, B. Satpati, P. V. Satyam, B. N. Dev and D. D. Sarma, J. Mater. Chem., 2004, 14, 661–668 RSC.
- C. Lemire, R. Meyer, S. Shaikhutdinov and H. J. Freund, Angew. Chem., Int. Ed., 2004, 43, 118–121 CrossRef PubMed.
- L. Yin, Y. Wang, G. Pang, Y. Koltypin and A. Gedanken, J. Colloid Interface Sci., 2002, 246, 78–84 CrossRef CAS PubMed.
- S. Sudo, S. Nagata, K. Kokado and K. Sada, Chem. Lett., 2014, 43, 1207–1209 CrossRef CAS.
- S. Penner, B. Klotzer, B. Jenewein, F. Klauser, X. Liu and E. Bertel, Thin Solid Films, 2008, 516, 4742–4749 CrossRef CAS.
- A. V. Dijken, E. A. Meulenkamp, D. Vanmaekelbergh and A. Meijerink, J. Lumin., 2000, 90, 123–128 CrossRef.
- L. Brus, J. Phys. Chem., 1986, 90, 2555–2560 CrossRef CAS.
- S. Ajaikumar and A. Pandurangan, Appl. Catal., A, 2009, 357, 184–192 CrossRef CAS.
- J. Sun, Q. Kan, Z. Li, G. Yu, H. Liu, X. Yang, Q. Huo and J. Guan, RSC Adv., 2014, 4, 2310–2317 RSC.
- N. Narender, K. V. V. K. Mohan, S. J. Lulkarni and I. A. K. Reddy, Catal. Commun., 2006, 7, 583–588 CrossRef CAS.
Footnote |
† Electronic supplementary information (ESI) available: Histogram of diameter, Brus equation, fluorescence and reflectance spectra. See DOI: 10.1039/c6ra26085c |
|
This journal is © The Royal Society of Chemistry 2017 |
Click here to see how this site uses Cookies. View our privacy policy here.