DOI:
10.1039/C7RA02758C
(Paper)
RSC Adv., 2017,
7, 27243-27247
Highly efficient synthesis of unsymmetrical 1,3-diynes from organoalane reagents and alkynyl bromides mediated by a nickel catalyst†
Received
7th March 2017
, Accepted 18th May 2017
First published on 23rd May 2017
Abstract
Highly efficient and simple cross-coupling reactions of alkynylbromides with organoalane reagents for the synthesis of unsymmetrical 1,3-diynes derivatives using Ni(OAc)2 (2–5 mol%)/(o-furyl)3P (4–10 mol%) as a catalyst are reported. Excellent yields (up to 94%) were obtained for a wide range of substrates at rt or 60 °C for 2–3 h in Et2O or toluene.
Diynes have been widely used in organic synthesis due to their significance as building blocks.1 Amongst them, conjugated 1,3-diynes have significant applications in the preparation of natural products,2 pharmaceuticals,3 π-conjugated acetylenic polymers,4 advanced materials5 and heterocyclic compounds,6 as well as in electronic and optical materials,7 and elsewhere.8 In addition, 1,3-diynes are common structural motifs found in pharmaceutically and biologically active compounds, which are known to have antifungal,9 antibacterial,10 anti-inflammatory,11 anti-HIV,12 and anticancer properties.13 Thus, their synthesis and applications has attracted a great amount of attention over the past few decades. Since the discovery of the Glaser homo-coupling reaction of terminal alkynes in 1869,14 a great amount of attention has been given to the development of new and efficient procedures for the synthesis of conjugated 1,3-diyne derivatives. Recently, a variety of processes have been developed for the preparation of conjugated 1,3-diynes, typical synthetic protocols including the cross-coupling reactions of 1-haloalkynes with terminal alkynes,15 cross-coupling reactions of terminal alkynes,16 cross-coupling reaction of terminal alkynes with 1,2-dihaloalkenes,17 cross-coupling of alkynylsilanes with haloalkynes,18 conjugated diynones decarbonylation reaction,19 cross-coupling reactions of aryl iodides and alkynyl acid,20 oxidative coupling of alkynyl Grignard reagents,21 oxidative decarboxylative homo-coupling of alkynyl acids,22 oxidative homo-coupling reactions of terminal alkynes with the oxidants,23 homo-coupling of alkynyltellurides,24 haloalkynes,25 and alkynylsilanes.26
Despite that, these efforts have provided alternative methods for the synthesis of conjugated 1,3-dyines. However, these reactions still suffer from excess oxidants, excess bases, cocatalysts, high temperature, relatively long reaction times, and the special reaction medium. The development of more efficient and atom economical approaches for the synthesis of conjugated 1,3-diynes remains as desirable work. In addition to the above reagents, alkynylaluminum reagents have been extensively used as nucleophiles for organic reactions.27
In recent times, metal-catalyzed cross-coupling reactions of electrophiles with alkynylmetallic reagents have provided an alternative route for the preparation of alkyne compounds.28 Previous studies show that organoalanes reagents are a highly efficient nucleophiles for cross-coupling reactions with aromatic halides29 or benzylic halides,30 and investigations have demonstrated that nickel is a good catalytic metal.31 To continue our efforts in developing coupling reactions using reactive organometallic reagents,29,30,32 we report a novel nickel(II)-catalyzed cross-coupling of alkynylhalides with alkynylaluminum reagents at ambient temperature or 60 °C in a short time with good isolated yield for the synthesis of unsymmetrical conjugated 1,3-diynes (Scheme 1).
 |
| Scheme 1 Metal-catalyzed coupling reactions of 1-haloalkynes derivatives with organoalanes nucleophiles. | |
In order to optimize the reaction conditions, effects of phosphine ligand, nickel source, reaction time, solvent, the amount of organoalanes reagents, and the molar ratio of metal to ligand on the cross-coupling reaction were investigated using alkynylaluminum and alkynyl bromide as a model system. The reaction of diethyl(2-phenylethynyl)aluminum (C6H5C
CAlEt2) (1a) with 1-(2-bromo-ethynyl)-4-methylbenzene (p-MeC6H5C
CBr) (2a) using a nickel catalyst of phosphine (eqn (1)) was first examined. Under the optimized conditions of 2 mol% Ni(OAc)2 and 4 mol% P(o-furyl)3 conducting in diethyl ether at room temperature over 2 h, producing 3aa in 87% isolated yield (ESI: Tables S1 and S2†).
|
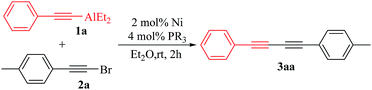 | (1) |
Generalities of the catalytic system were examined with various 1-bromoalkynes derivatives (eqn (2)), and the results were summarized in Table 1. As shown in Table 1, various 1-bromoalkynes derivatives bearing electron-withdrawing or electron-donating substituents were smoothly coupled to give the corresponding unsymmetrical conjugated 1,3-diynes 3 in 64–94% isolated yield (Table 1, entries 1–14). The cross-coupling reaction with 1-bromoalkynes bearing an electron-withdrawing group, such as fluoro, chloro, bromo and trifluoromethyl groups, or electron-donating group, such as methyl, ethyl, propyl and tertbutyl groups, produced the conjugated products 1,3-diyne in good to excellent yield (Table 1, entries 1–12). In particular, the cross-coupling of 1-bromo-4-(2-bromoethynyl) benzene (2g) with diethyl-(2-phenylethynyl)aluminum (1a) could also be selectively obtained the unsymmetrical conjugated 1,3-diynes 3ag in 74% isolated yield (Table 1, entry 7). However, the cross-coupling reaction of diethyl-(2-phenylethynyl)aluminum (1a) with 1-(2-bromoethynyl)-4-methoxybenzene (4-MeOC6H5C
CBr) (2f) only gave products of unsymmetrical conjugated 1,3-diyne 3af in 64% isolated yield (Table 1, entry 6) potentially due to the 1-(2-bromoethynyl)-4-methoxybenzene was being decreased by methoxyl group. More importantly, 2-(2-bromoethynyl)pyridine and 2-(2-bromoethynyl)thiophene reagents were also effective substrates for this reaction, giving the corresponding coupling products of unsymmetrical conjugated 1,3-diynes 3am and 3an in 83% and 94% isolated yield, respectively (Table 1, entries 13 and 14). Unfortunately, cross-coupling reaction of 4-(2-bromoethynyl)-benzonitrile (2o) (p-CNC6H5C
CBr) and diethyl-(2-phenylethynyl)-aluminum (1a) (C6H5C
CAlEt2) only gave products of unsymmetrical conjugated 1,3-diyne 3ao in 5% yield (Table 1, entries 15). While, coupling of methyl 4-(2-bromoethynyl)benzoate (2p) (p-CH3OOCC6H5C
CBr) could not produce the target compound (Table 1, entries 16).
Table 1 Nickel-catalyzed cross-coupling reactions of C6H5C
CAlEt2 (1a) with various 1-bromoalkynes (2)a
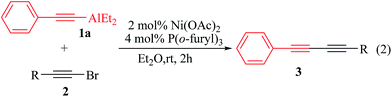
|
Entry |
1-Bromoalkynes 2 |
Product 3 |
Yieldb (%) |
1a/2 = 1.0/0.5 mmol; 2 mL Et2O; room temperature; 2 h. Isolated yield. |
1 |
4-Me-Ph (2a) |
3aa |
87 |
2 |
4-Et-Ph (2b) |
3ab |
85 |
3 |
4-Propyl-Ph (2c) |
3ac |
86 |
4 |
4-tBu-Ph (2d) |
3ad |
84 |
5 |
3-Me-Ph (2e) |
3ae |
81 |
6 |
4-MeO-Ph (2f) |
3af |
64 |
7 |
4-Br-Ph (2g) |
3ag |
74 |
8 |
4-Cl-Ph (2h) |
3ah |
82 |
9 |
4-F-Ph (2i) |
3ai |
90 |
10 |
3-F-Ph (2j) |
3aj |
93 |
11 |
2-F-Ph (2k) |
3ak |
86 |
12 |
4-CF3-Ph (2l) |
3al |
92 |
13 |
2-Pyridinyl (2m) |
3am |
94 |
14 |
2-Thienyl (2n) |
3an |
83 |
15 |
4-CN-Ph (2o) |
3ao |
<5% |
16 |
4-CH3OOC-Ph (2p) |
3ap |
0 |
We subsequently investigated cross-coupling reactions of substituted aryl alkynylaluminum reagents with various 1-bromoalkynes. However, the cross-coupling reaction of diethyl(2-(4-methoxy phenyl)-ethynyl)aluminum (4-MeOC
CAlEt2) (1b) with 1-(2-bromo-ethynyl)-benzene (2o) employing the catalyst of 2 mol% Ni(OAc)2 and 4 mol% P(o-furyl)3 yielded coupling products of unsymmetrical conjugated 1,3-diyne 3bo with only a 62% isolated yield. Therefore, the reaction conditions were re-tuned, and the best performed catalyst was found to be 5 mol% Ni(OAc)2 and 10 mol% P(o-furyl)3 while the reaction was conducting in toluene at 60 °C for 3 h (ESI Table S3†), furnishing 3bo with 75% isolated yield.
The reaction scope was further explored on substrates of R′C
CAlEt2 (R′ = 4-MeOPh (1b) or 4-FPh (1c), 4-MePh (1d)) and various 1-bromoalkynes using 5 mol% Ni(OAc)2 and 10 mol% P(o-furyl)3 conducting in toluene at 60 °C for 3 h (eqn (3)), and results are summarized in Table 2. Satisfactory application scope was demonstrated by this section of experiment. The cross-coupling reaction can be applied to C(sp)–C(sp) bond formations, affording the coupling products unsymmetrical conjugated 1,3-diynes in 51–80% isolated yield (Table 2, 1–15). Cross-coupling reactions of substituted aryl alkynylaluminum reagents 1b or 1c or 1d with 1-bromoalkyne reagents containing different functional groups such as alkyl, halides (2a, 2g, 2h, 2i, 2j, 2k, 2l) could potentially couple, giving the corresponding unsymmetrical conjugated 1,3-diynes moderate to good isolated yields (Table 2, entries 1–4, 7, 8, 10–14). Furthermore, 2-(2-bromoethynyl)thiophene (2n) reacted successfully with diethyl(2-(4-methoxyphenyl)ethynyl)aluminum (4-MeOPhC
C–AlEt2) (1b) to provide unsymmetrical conjugated 1,3-diyne 3bn with 74% isolated yield (Table 2, entry 5). The 2-(4-p-tolylbuta-1,3-diynyl)pyridine (3dm) resulting from 2-(2-bromo-ethynyl)pyridine (2m) and diethyl(2-p-tolyl-ethynyl)aluminum (4-MePhC
CAlEt2) (1d) was obtained with 72% isolated yield (Table 2, entry 15). While, the cross-coupling reaction of diethyl(2-(4-fluorophenyl)-ethynyl) aluminium (4-FPhC
CAlEt2) (1c) with 1-bromo-hept-1-yne (2p) gave the corresponding unsymmetrical conjugated 1,3-diyne 3cp with a 51% isolated yield (Table 2, entry 9). Unfortunately, cross-coupling of 1-(2-bromoethynyl)-4-methylbenzene (2a) (p-CH3C6H5C
CBr) and diethyl(2-(4-methylbenzoate)ethynyl) aluminium (4-MeOOCPhC
CAlEt2) (1e) could not produce the coupling product (Table 2, entries 16).
Table 2 Cross-coupling reactions of substituted aryl organoalanes reagents ArC
CAlEt2 (1) with various 1-bromoalkynes (2)a
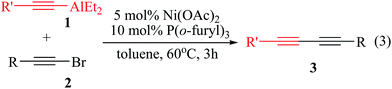
|
Entry |
1 R′ |
2 R |
Product |
Yieldb (%) |
1/2 = 1.0/0.5 mmol; 2 mL toluene; 60 °C; 3 h. Isolated yield. |
1 |
4-MeOPh (1b) |
4-MePh (2a) |
3ba |
62 |
2 |
4-MeOPh (1b) |
4-ClPh (2h) |
3bh |
72 |
3 |
4-MeOPh (1b) |
4-FPh (2i) |
3bi |
71 |
4 |
4-MeOPh (1b) |
3-FPh (2j) |
3bj |
72 |
5 |
4-MeOPh (1b) |
2-Thienyl (2n) |
3bn |
74 |
6 |
4-MeOPh (1b) |
Ph (2o) |
3bo |
75 |
7 |
4-FPh (1c) |
3-FPh (2j) |
3cj |
55 |
8 |
4-FPh (1c) |
2-FPh (2k) |
3ck |
53 |
9 |
4-FPh (1c) |
n-Pentyl (2p) |
3cp |
51 |
10 |
4-MePh (1d) |
4-BrPh (2g) |
3dg |
68 |
11 |
4-MePh (1d) |
4-FPh (2i) |
3di |
62 |
12 |
4-MePh (1d) |
3-FPh (2j) |
3dj |
73 |
13 |
4-MePh (1d) |
2-FPh (2k) |
3dk |
80 |
14 |
4-MePh (1d) |
4-CF3Ph (2l) |
3dl |
75 |
15 |
4-MePh (1d) |
2-Pyridinyl (2m) |
3dm |
72 |
16 |
4-MeOOCPh (1e) |
4-MePh (2a) |
3af |
0 |
The cross-coupling reactions of aliphatic alkynylaluminum reagents with various 1-bromoalkynes was also investigated under the same catalytic system. The unsymmetrical conjugated 1,3-diyne 3fo was obtained with 52% isolated yield when 1-(2-bromoethynyl)-benzene (2o) coupled with the TMSC
CAlEt2 (1f) nucleophile. However, the cross-coupling reaction of 1-(2-bromoethynyl)-benzene (2o) coupled with the TMSC
CAlEt2 (1f) favoured a formation of the unsymmetrical conjugated 1,3-diyne 3fo with 80% isolated yield in DME at room temperature for 3 h (ESI Table S4†). So, the reaction scope was further explored on substrates of TMSC
CAlEt2 (1f) and various 1-bromoalkynes using 5 mol% Ni(OAc)2 and 10 mol% P(o-furyl)3 conducting in DME at room temperature for 3 h (eqn (4)), and results are summarized in Table 3. As shown in Table 3, various 1-bromoalkynes derivatives bearing electron-withdrawing or electron-donating substituents were smoothly coupled to give the corresponding unsymmetrical conjugated 1,3-diynes 3 in 67–80% isolated yield (Table 3, entries 1–6). The cross-coupling reaction with 1-bromoalkynes bearing an electron-withdrawing group, such as fluoro and trifluoromethyl groups, or electron-donating group, such as methoxyl and tertbutyl groups, produced the conjugated products 1,3-diyne in good isolated yield (Table 3, entries 2–5). In particular, the cross-coupling of 2-(2-bromoethynyl)naphthalene (2q) with TMSC
CAlEt2 (1f) could also be obtained the conjugated 1,3-diynes 3fq in 74% isolated yield (Table 3, entry 6).
Table 3 Cross-coupling reactions of organoalanes reagents TMSC
CAlEt2 (1f) with various 1-bromoalkynes (2)a
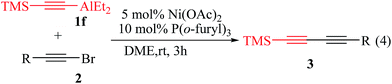
|
Entry |
2 R |
Product |
Yieldb (%) |
1/2 = 1.0/0.5 mmol; 2 mL DME; room temperature; 3 h. Isolated yield. |
1 |
Ph (2o) |
3fo |
80 |
2 |
4-tBu-Ph (2d) |
3fd |
74 |
3 |
4-MeOPh (2f) |
3ff |
76 |
4 |
4-FPh (2i) |
3fi |
72 |
5 |
4-CF3Ph (2l) |
3fl |
67 |
6 |
2-Naphthyl (2q) |
3fq |
74 |
The effect of the alkylhalides and dummy ligand of organoalane reagents on the reaction were investigated, and the results were summarized in Table 4. As shown in Table 4, cross-coupling reaction of dimethyl-(2-phenylethynyl)-aluminum (1g) (C6H5C
CAlMe2) with p-CH3C6H5C
CBr (2a) gave product of unsymmetrical conjugated 1,3-diyne 3aa in 68% isolated yield (Table 4, entry 1). The coupling reaction of propargyl iodide (2q) with diethyl-(2-phenylethynyl)-aluminum (1a) (C6H5C
CAlEt2) gave product of unsymmetrical conjugated 1,3-diyne 3aa in a 75% isolated yield (Table 4, entry 2). While, coupling of propargyl chloride (2r) gave coupling product 3aa in only 48% isolated yield (Table 4, entry 3). Gratifyingly, 70% isolated yield of product 3aa was obtained using tri(2-phenylethynyl)-aluminum (1h) as nucleophiles (Table 4, entry 4). The results showed that the different alkylhalides and dummy ligand of organoalane reagents are compatible with this procedure.
Table 4 The effect of the alkylhalides and dummy ligand of organoalane reagents on the reactiona
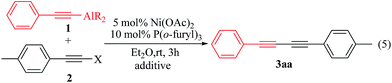
|
Entry |
R (1) |
X (2) |
Product |
Yieldb (%) |
1/2 = 1.0/0.5 mmol; 2 mL Et2O; room temperature; 3 h. Isolated yield. |
1 |
Me (1g) |
Br (2a) |
3aa |
68 |
2 |
Et (1a) |
I (2q) |
3aa |
75 |
3 |
Et (1a) |
Cl (2r) |
3aa |
48 |
4 |
PhC C– (1h) |
Br (2a) |
3aa |
70 |
While a precise understanding of the reaction mechanism awaits further study, a plausible catalytic pathway is proposed in Scheme 2. The first step is the oxidative addition of 1-bromoalkyne (1) to Ni(0) phosphine complex (4) (which in turn from Ni(OAc)2 and R′C
CAlEt2 (2) reagents) that forms the organonickel(II) bromide intermediate (5). Transmetalation of R′C
CAlEt2 (2) with 5 gives alkyne-alkynenickelium(II) intermediate (6) and Et2AlBr. Finally, complex 6 under goes reductive elimination to afford the desired cross-coupling product 3 and regenerate the active Ni(0) species for the next catalytic cycle.
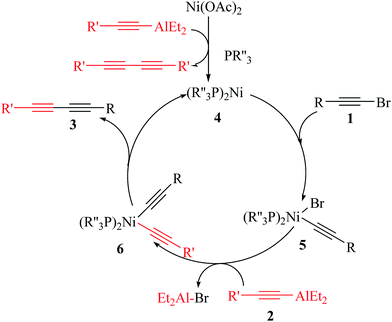 |
| Scheme 2 The proposed catalytic cycle for the formation of unsymmetrical conjugated 1,3-diyne 3. | |
Conclusions
In conclusion, a novel nickel-catalyzed cross-coupling reaction of 1-bromoalkynes with alkynylaluminum reagents is reported. A variety of 1-bromoalkynes with aryl, heterocycloyl, or alkyl group can be efficiently coupled to give the corresponding coupling products unsymmetrical conjugated 1,3-diynes in good yield and with good functional group compatibility. Moreover, the ready availability of the starting materials, the mild reaction conditions, and the simplicity of the operations involved are additional features making the methodology could therefore serve as a complementary option in the field of conjugated 1,3-diyne synthesis. A possible catalytic cycle was proposed to explain the formation of the products conjugated 1,3-diynes. Further application of these conjugated 1,3-diynes in organic synthesis are underway.
Acknowledgements
The authors are grateful to the Sichuan Provincial Department of science and technology support program (No. 2015NZ0033), and the Postgraduate Degree Construction Project of Southwest University for Nationalities (No. CX2016SZ063) for financial support.
Notes and references
-
(a) Y. Matsuda, S. Naoe, S. Oishi, N. Fujii and H. Ohno, Chem.–Eur. J., 2015, 21, 1463 CrossRef CAS PubMed;
(b) Y. G. Zhu and Y. A. Shi, Org. Biomol. Chem., 2013, 11, 7451 RSC.
-
(a) A. L. K. Shi Shun and R. R. Tykwinski, Angew. Chem., Int. Ed., 2006, 45, 1034 CrossRef PubMed;
(b) H. Yun, T. C. Chou, H. J. Dong, Y. Tian, Y. M. Li and S. J. Danishefsky, J. Org. Chem., 2005, 70, 10375 CrossRef CAS PubMed.
-
(a) M. L. Lerch, M. K. Harper and D. J. Faulkner, J. Nat. Prod., 2003, 66, 667 CrossRef CAS PubMed;
(b) K. C. Nicolau, P. G. Bulger and D. Sarlah, Angew. Chem., Int. Ed., 2005, 44, 4490 CrossRef PubMed.
-
(a) B. J. Morgan, X. Xie, P. W. Phuan and M. C. Kozlowski, J. Org. Chem., 2007, 72, 6171 CrossRef CAS PubMed;
(b) F. Babudri, D. Colangiuli, P. A. Di Lorenzo, G. M. Farinola, O. H. Omar and F. Naso, Chem. Commun., 2003, 130 RSC.
-
(a) Acetylene Chemistry: Chemistry, Biology and Material Science, ed. F. Diederich, P. J. Stang and R. R. Tykwinski, Wiley-VCH GmbH KGaA, Weinheim, Germany, 2005 Search PubMed;
(b) M. Gholami and R. R. Tykwinski, Chem. Rev., 2006, 106, 4997 CrossRef CAS PubMed.
-
(a) V. Lavallo, G. D. Frey, B. Donnadieu, M. Soleilhavoup and G. Bertrand, Angew. Chem., Int. Ed., 2008, 47, 5224 CrossRef CAS PubMed;
(b) T. Matsuda, S. Kadowaki and M. Murakami, Chem. Commun., 2007, 2627 RSC.
-
(a) J. A. Marsden and M. M. Haley, J. Org. Chem., 2005, 70, 10213 CrossRef CAS PubMed;
(b) S. Eisler, A. D. Slepkov, E. Elliott, T. Luu, R. McDonald, F. A. Hegmann and R. R. Tykwinski, J. Am. Chem. Soc., 2005, 127, 2666 CrossRef CAS PubMed.
-
(a) M. B. Nielsen and F. Diederich, Chem. Rev., 2005, 105, 1837 CrossRef CAS PubMed;
(b) J. Z. Liu, J. W. Y. Lam and B. Z. Tang, Chem. Rev., 2009, 109, 5799 CrossRef CAS PubMed.
- A. Stütz, Angew. Chem., Int. Ed., 1987, 26, 320 CrossRef.
- D. Lechner, M. Stavri, M. Oluwatuyi, R. Perda-Miranda and S. Gibbons, Phytochemistry, 2004, 65, 331 CrossRef CAS PubMed.
-
(a) R. Schmidt, R. Thorwirth, T. Szuppa, A. Stolle, B. Ondruschka and H. Hopf, Chem.–Eur. J., 2011, 17, 8129 CrossRef CAS PubMed;
(b) R. A. Xiao, R. Y. Yao and M. Z. Cai, Eur. J. Org. Chem., 2012, 22, 4178 CrossRef;
(c) A. Narani, R. K. Marella, P. Ramudu, K. S. R. Rao and D. R. Burri, RSC Adv., 2014, 4, 3774 RSC.
- M. L. Lerch, M. K. Harper and D. J. Faulkner, J. Nat. Prod., 2003, 66, 667 CrossRef CAS PubMed.
-
(a) S. Morandi, F. Pellati, S. Benvenuti and F. Prati, Tetrahedron, 2008, 64, 6324 CrossRef CAS;
(b) S. F. Mayer, A. Steinreiber, R. V. A. Orru and K. Faber, J. Org. Chem., 2002, 67, 9115 CrossRef CAS PubMed.
- C. Glaser, Ber. Dtsch. Chem. Ges., 1869, 2, 422 CrossRef.
-
(a) H. L. Li, L. L. Wang, M. Yang and Y. X. Qi, Catal. Commun., 2012, 17, 179 CrossRef CAS;
(b) D. Saha, T. Chatterjee, M. Mukherjee and B. C. Ranu, J. Org. Chem., 2012, 77, 9379 CrossRef CAS PubMed;
(c) W. Shi, Y. D. Luo, X. C. Luo, L. Chao, H. Zhang, J. Wang and A. W. Lei, J. Am. Chem. Soc., 2008, 130, 14713 CrossRef CAS PubMed;
(d) Z. W. Chen, H. F. Jiang, A. Z. Wang and S. R. Yang, J. Org. Chem., 2010, 75, 6700 CrossRef CAS PubMed;
(e) S. Ahammed, D. Kundu and B. C. Ranu, J. Org. Chem., 2014, 79, 7391 CrossRef CAS PubMed.
-
(a) J. R. Suárez, D. Collado-Sanz, D. J. Cárdenas and J. L. Chiara, J. Org. Chem., 2015, 80, 1098 CrossRef PubMed;
(b) M. Nasr-Esfahani, I. Mohammadpoor-Baltork, A. R. Khosropour, M. Moghadam, V. Mirkhani, S. Tangestaninejad, V. Agabekov and H. A. Rudbaria, RSC Adv., 2014, 4, 14291 RSC;
(c) Z. Y. Ma, X. Y. Wang, S. Y. Wei, H. L. Yang, F. W. Zhang, P. Wang, M. Xie and J. T. Ma, Catal. Commun., 2013, 39, 24 CrossRef CAS.
- M. L. N. Rao, P. Dasgupta, B. S. Ramakrishna and V. N. Murty, Tetrahedron Lett., 2014, 55, 3529 CrossRef CAS.
- Y. Nishihara, K. Ikegashira, A. Mori and T. Hiyama, Tetrahedron Lett., 1998, 39, 4075 CrossRef CAS.
- A. Dermenci, R. E. Whittaker and G. Dong, Org. Lett., 2013, 15, 2242 CrossRef CAS PubMed.
-
(a) Y. Kim, A. Park, K. Park and S. Lee, Tetrahedron Lett., 2011, 52, 1766 CrossRef CAS;
(b) J. Moon, M. Jeong, H. Nam, J. Ju, J. H. Moon, H. M. Jung and S. Lee, Org. Lett., 2008, 10, 945 CrossRef CAS PubMed;
(c) J. Moon, M. Jang and S. Lee, J. Org. Chem., 2009, 74, 1403 CrossRef CAS PubMed;
(d) K. Park, G. Bae, J. Moon, J. Choe, K. H. Song and S. Lee, J. Org. Chem., 2010, 75, 6244 CrossRef CAS PubMed;
(e) K. Pak, G. Bae, A. Park, Y. Kim, J. Choe, K. H. Song and S. Lee, Tetrahedron Lett., 2011, 52, 576 CrossRef.
-
(a) Y. G. Zhu, T. Xiong, W. Y. Han and Y. A. Shi, Org. Lett., 2014, 16, 6144 CrossRef CAS PubMed;
(b) G. Cahiez, C. Chaboche, F. Mahuteau-Betzer and M. Ahr, Org. Lett., 2005, 7, 1943 CrossRef CAS PubMed;
(c) A. Krasovskiy, A. Tishkov, V. del Amo, H. Mayr and P. Knochel, Angew. Chem., Int. Ed., 2006, 45, 5010 CrossRef CAS PubMed.
- D. X. Liu, F. L. Li, H. X. Li, J. Gao and J. P. Lang, Tetrahedron, 2014, 70, 2416 CrossRef CAS.
- For selected recent reports on the oxidative homocoupling reactions of terminal alkynes with the oxidants, see:
(a) H. L. Li, M. Yang, X. Zhang, L. Yan, J. Li and Y. X. Qi, New J. Chem., 2013, 37, 1343 RSC;
(b) F. Alonso and M. Yus, ACS Catal., 2012, 2, 1441 CrossRef CAS;
(c) K. Yin, C. J. Li, J. Li and X. S. Jia, Green Chem., 2011, 13, 591 RSC;
(d) R. A. Xiao, R. Y. Yao and M. Z. Cai, Eur. J. Org. Chem., 2012, 4178 CrossRef CAS;
(e) S. L. Zhang, X. Y. Liu and T. Q. Wang, Adv. Synth. Catal., 2011, 353, 1463 CrossRef CAS;
(f) W. Y. Yin, C. He, M. Chen, H. Zhang and A. W. Lei, Org. Lett., 2009, 11, 709 CrossRef CAS PubMed;
(g) X. Meng, C. B. Li, B. C. Han, T. S. Wang and B. H. Chen, Tetrahedron, 2010, 66, 4029 CrossRef CAS;
(h) Y. G. Zhu and Y. A. Shi, Org. Biomol. Chem., 2013, 11, 7451 RSC;
(i) J. R. Suárez, D. Collado-Sanz, D. J. Cárdenas and J. L. Chiara, J. Org. Chem., 2015, 80, 1098 CrossRef PubMed;
(j) K. Fang, M. H. Xie, Z. N. Zhang, P. Ning and G. Y. Shu, Tetrahedron Lett., 2013, 54, 3819 CrossRef CAS.
- F. V. Singh, M. F. Z. J. Amaral and H. A. Stefani, Tetrahedron Lett., 2009, 50, 2636 CrossRef CAS.
-
(a) K. Osowska, T. Lis and S. Szafert, Eur. J. Org. Chem., 2008, 27, 4598 CrossRef;
(b) Z. Chen, H. Jiang, A. Wang and S. Yang, J. Org. Chem., 2010, 75, 6700 CrossRef CAS PubMed.
- Y. Nishihara, K. Ikegashira, K. Hirabayashi, J. Ando, A. Mori and T. Hiyama, J. Org. Chem., 2000, 65, 1780 CrossRef CAS PubMed.
-
(a) B. Shrestha, S. Thapa, S. K. Gurung, R. A. S. Pike and R. Giri, J. Org. Chem., 2016, 81, 787 CrossRef CAS PubMed;
(b) D. F. Crépin and J. P. A. Harrity, Org. Lett., 2013, 15, 4222 CrossRef PubMed;
(c) O. V. Larionov and E. J. Corey, Org. Lett., 2010, 12, 300 CrossRef CAS PubMed;
(d) W. Dávila, W. Torres and J. A. Prieto, Tetrahedron, 2007, 63, 8218 CrossRef PubMed;
(e) M. Sasaki, K. Tanino and M. Miyashita, Org. Lett., 2001, 3, 1765 CrossRef CAS PubMed;
(f) M. Sasaki, M. Miyazawa, K. Tanino and M. Miyashita, Tetrahedron Lett., 1999, 40, 9267 CrossRef CAS.
-
(a) B. M. Wang, M. Bonin and L. Micouin, Org. Lett., 2004, 6, 3481 CrossRef CAS PubMed;
(b) R. Chinchilla and C. Nàjera, Chem. Rev., 2007, 107, 874 CrossRef CAS PubMed;
(c) E.-I. Negishi and L. Anastasia, Chem. Rev., 2003, 103, 1979 CrossRef CAS PubMed;
(d) J. Kessabi, R. Beaudegnies, P. M Jung, B. Martin, F. Montel and S. Wendeborn, Synthesis, 2008, 4, 655 Search PubMed;
(e) H. Tanaka and Y. Shishido, Bioorg. Med. Chem. Lett., 2007, 17, 6079 CrossRef CAS PubMed.
-
(a) D. B. Biradar and H. M. Gau, Chem. Commun., 2011, 47, 10467 RSC;
(b) W. T. Shu, S. L. Zhou and H. M. Gau, Synthesis, 2009, 3, 4075 Search PubMed;
(c) S. L. Ku, X. P. Hui, C. A. Chen, Y. Y. Kuo and H. M. Gau, Chem. Commun., 2007, 3847 RSC.
- D. B. Biradar and H. M. Gau, Org. Biomol. Chem., 2012, 10, 4243 CAS.
-
(a) J. Caeiro, J. P. Sestelo and L. A. S arandeses, Chem.–Eur. J., 2008, 14, 741 CrossRef CAS PubMed;
(b) I. Koyama, T. Kurahashi and S. Matsubara, J. Am. Chem. Soc., 2009, 131, 1350 CrossRef CAS PubMed;
(c) Y. Shi, J. Huang, Y. F. Yang, L. Y. Wu, Y. N. Niu, P. F. Huo, X. Y. Liu and Y. M. Liang, Adv. Synth. Catal., 2009, 351, 141 CrossRef CAS;
(d) J. Terao, F. Bando and N. Kambe, Chem. Commun., 2009, 7336 RSC;
(e) S. Sako, T. Kurahashi and S. Matsubara, Chem. Commun., 2011, 47, 6150 RSC.
-
(a) Q. H. Li, J. Y. Jeng and H. M. Gau, Eur. J. Org. Chem., 2014, 31, 7531 Search PubMed;
(b) Q. H. Li, J. W. Liao, Y. L. Huang, R. T. Chiang and H. M. Gau, Org. Biomol. Chem., 2014, 12, 7634 RSC;
(c) Q. H. Li and H. M. Gau, Synlett, 2012, 5, 747 CrossRef;
(d) Q. H. Li, Y. Ding and X. J. Yang, Chin. Chem. Lett., 2014, 25, 1296 CrossRef CAS.
Footnote |
† Electronic supplementary information (ESI) available. See DOI: 10.1039/c7ra02758c |
|
This journal is © The Royal Society of Chemistry 2017 |
Click here to see how this site uses Cookies. View our privacy policy here.